One of the most prevalent tools in the field of bioelectronics is the organic electrochemical transistor (OECT). Due to its mixed (ionic and electronic) conduction properties and the flexibility of the material in its configuration, mechanical, and interfacial properties, OECTs lend themselves to a diverse set of biological applications. They have been used to monitor important cell metabolites like lactate and cholesterol1 2 and are capable of operating in complex biological media like saliva and sweat3 4. When coupled with bioactive material like antibodies, OECTs can be used to detect specific antigen targets quickly and with high sensitivity using unprocessed biological samples5. Further, being a ‘soft’ electronic compatible with aqueous media with low power consumption, OECTs can function as wearable devices, finding themselves inside the cortex monitoring long-term brain function6 and as wearable electrophysiological sensor skin patches7. With such a diverse set of applications spread across many fields, it can be challenging to characterize and optimize the components of an OECT. In particular, the mixed conduction properties of an OECT greatly impact the overall transduction of a device. Electrochemical-Quartz Crystal Microbalance with Dissipation (EQCM-)D, can provide key mechanistic insights into the ionic conduction properties under in situ conditions, along with quantification of molecular interactions occurring at specialized OECT interfaces.
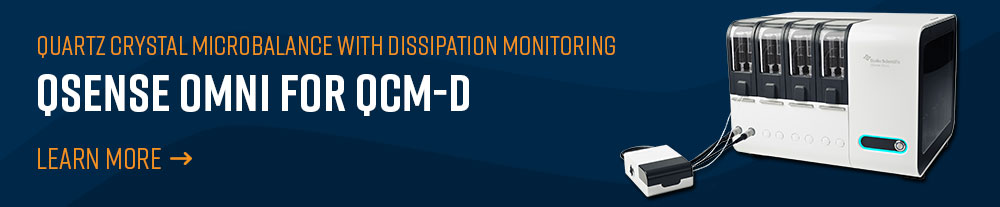
How Do Organic Electrochemical Transistors Work?
As the name suggests, an OECT operates as a transistor, functioning as an on/off switch while amplifying an ionic signal. Figure 1 illustrates the basic components of an OECT, made up of a gate, source, and drain electrodes, with a channel between the source and drain, and an electrolyte between the gate and the channel. The channel is made up of a mixed conducting polymer, referred to as an organic mixed ionic electronic conductor or OMIEC film. The OMEIC film allows the transport of both holes/electrons and ions and affects the current flow from the source to the drain electrodes. This current flow in the channel is the main signal of the OECT. The bulk conductivity of the channel can be modulated by injecting ions from the electrolyte, thereby directly affecting the current flow. Thus, the ionic flux from the electrolyte into the channel is converted into electronic signals, amplifying the ionic signal in the process. In achieving a large ionic amplification with a quick response, the OECT can be utilized for low-concentration analyte biosensing.
Operation of OECTs
In the typical operation of an OECT, a voltage is applied across the source and the drain (VDS) while the current that flows through the channel is monitored. This current is a function of the charge carriers and their speed. By applying a voltage to the gate electrode (VGS; usually with respect to the source which is grounded) ions in the electrolyte respond by flowing in or out of the channel. The direction in which ions flow and their effect on the channel current will depend on the intrinsic mixed-charged transport properties of the OMIEC channel film. These properties will influence which of the two operational modes the OECT will employ: accumulation mode or depletion mode.
If the OMIEC layer is undoped (lacks charge carriers) and has low conductivity, then the current between the source and the drain is initially minimal. Upon applying a gate voltage, ions are injected into the OMIEC channel, increasing the conductivity and therefore the current. This is an example of accumulation or enhancement mode, where the OECT switches from OFF to ON.
In depletion mode, the OMIEC channel uses a doped substrate with existing charge carriers and high conductivity, allowing significant current to flow from the source to the drain. When the gate voltage is applied here, ions from the electrolyte are introduced to the layer. Rather than increase the conductivity, the ions screen the doped charge carriers in the OMIEC channel, preventing current flow and switching the OECT from ON to OFF.
QCM-D: the Ultrasensitive In-situ Balance and Beyond
Quartz Crystal Microbalance with Dissipation monitoring (QCM-D) operates as an ultra-sensitive force balance, allowing the detection of sub-nanogram (<0.3 ng/cm2) changes in mass. The classic QCM technique takes advantage of the piezoelectric properties of quartz: utilizing a thin quartz wafer sandwiched between two electrodes, oscillating voltage is applied to the electrodes resulting in a shear resonance of the quartz. The frequency of this resonance is proportional to the mass of the sensor, allowing in situ and label-free monitoring of surface mass and thin film changes. Going beyond the mass, QCM with Dissipation monitoring further measures the decay of the resonant frequency, directly probing changes into the rigidity of the sensor surface.
The ability to detect both mass and softness changes of a film can provide information relevant to OECTs, like swelling, film thickness, ion intercalation, and degradation. As the sensor surface is intrinsically an electrode, it can be interfaced easily with a potentiostat to measure changes in mass/thickness/rigidity under a voltage bias. Figure 2 shows the experimental configuration of the electrochemical or eQCM-D setup.
Unique Position of eQCM-D for Characterizing Swelling in OECTs
The OMIEC layer is essential to the operation of an OECT, generally using an aqueous-based electrolyte as the interface between the channel and the gate. Unless it is a fully sold-state device, the OMIEC channel is commonly in the form of a hydrated polymer that is significantly conductive of ions. Thus, understanding the incorporation of both solvent and ions under operational conditions is necessary to optimize the OMIEC layer and improve the operation of an OECT.
To study this, eQCM-D is an adept method capable of measuring the dynamic swelling process of mixed polymeric conductors during voltage bias8. This can be done by coating a thin OMIEC layer on top of the electrically conductive QCM-D sensor surface. Using this coated sensor, the frequency (layer thickness) and dissipation (layer softness) are monitored in real-time across different periods of an OECT operation: introduction of solvent for initial swelling, introduction of ions in an electrolyte, and application of an electrochemical bias to simulate OECT function.
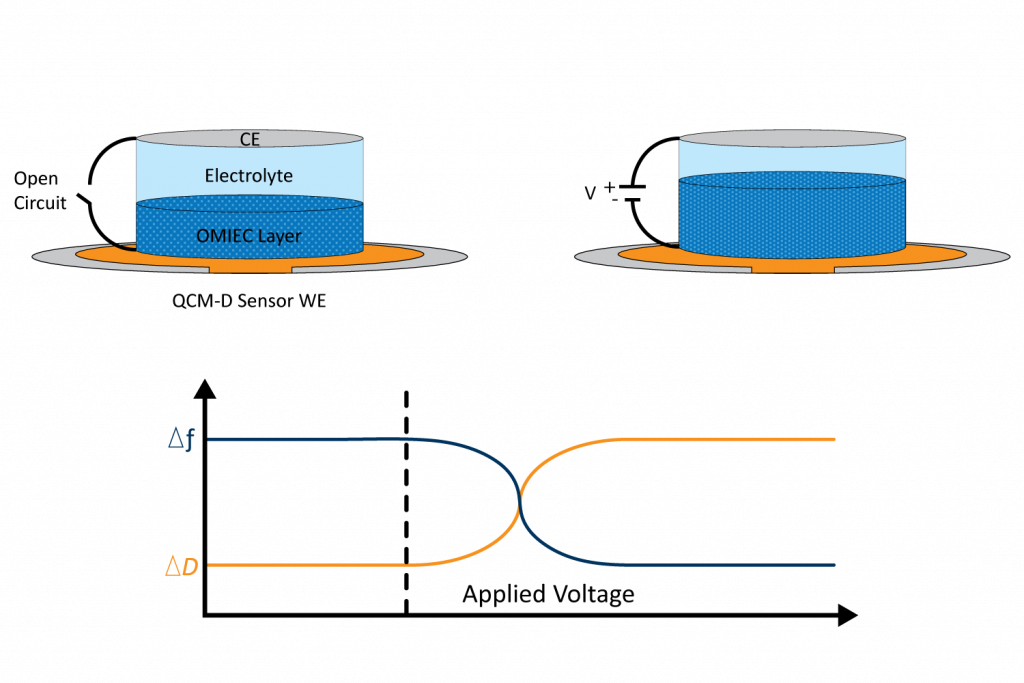
Figure 3 depicts an example eQCM-D measurement, where the frequency decreases and the dissipation increases in response to a negative voltage applied to the OMIEC-coated sensor: this signal response is typical of significant swelling. Combined with the charge data recorded from a potentiostat, the ion-to-electron efficiency can be determined along with the degree of solvent incorporation during different OECT operation modes. It has been found that a large quantity of water is incorporated during enhancement mode, which can increase ionic mobility but likewise decrease the charge mobility, impacting OECT efficiency8. Further, it is possible to model the QCM-D data (with the QSense DFind software using the Kelvin-Voigt model9) and reveal viscoelastic properties of the film.
Beyond the fundamental OMIEC layer understanding offered by eQCM-D, the QCM-D technique is highly effective at characterizing molecular binding and layer-building events. As specialized OECTs require various bioactive layers like antibodies, nucleotides, enzymes, or electroactive chemicals for sensing redox current, verifying that these layers are being incorporated into the sensor surfaces and participating in binding events is crucial. Measuring molecular interactions at a solid/liquid interface is what QCM-D excels at, and can directly help with building and configuring an OECT effectively 5.
Conclusion
With the high sensitivity and selectivity of OECTs, they are the go-to devices for biosensing and electrophysiological recording. However, there is still significant room for advancement with new materials, device configurations, and applications. For understanding one of the most important components of an OECT, the OMIEC channel, eQCM-D is a uniquely suited tool. eQCM-D gives the ability to characterize ion flux and swelling of the channel under an electrochemical bias, which can substantially impact OECT performance. Further, QCM-D is a great way to verify layer formation when functionalizing the various interfaces of an OECT for their specific application.
References
- Pappa, A.M., Ohayon, D., Giovannitti, A., Maria, I.P., Savva, A., Uguz, I., Rivnay, J., McCulloch, I., Owens, R.M., Inal, S. “Direct metabolite detection with an n-type accumulation mode organic electrochemical transistor.” Sci. Adv., vol. 4, no. 6, June. 2018. ↩︎
- Wustoni S., Hidalgo T. C., Hama A., Ohayon D., Savva A., Wei N., Wehbe N., Inal S., “In Situ Electrochemical Synthesis of a Conducting Polymer Composite for Multimetabolite Sensing.” Adv. Mater. Technol., vol. 5, no. 3, Jan. 2020. ↩︎
- Pappa A. M., Curto V. F., Braendlein M., Strakosas X., Donahue M. J., Fiocchi M., Malliaras G. G., Owens R. M., “Organic Transistor Arrays Integrated with Finger-Powered Microfluidics for Multianalyte Saliva Testing.” Adv. Healthcare Mater., vol. 5, no. 17, pp 2295-2302, Jul. 2016. ↩︎
- Currano L. J., Sage F. C., Hagedon M., Hamilton L., Patrone J., Gerasopoulos K., “Wearable Sensor System for Detection of Lactate in Sweat.” Sci. Rep., vol. 8, pp 1–11, Oct. 2018 ↩︎
- Guo, K., Wustoni, S., Koklu, A. et al. “Rapid single-molecule detection of COVID-19 and MERS antigens via nanobody-functionalized organic electrochemical transistors.” Nature Biomedical Engineering, vol. 5, no. 7, pp 666-677, May 2021. ↩︎
- Cea C., Spyropoulos G.D., Jastrzebska-Perfect P., Ferrero J.J., Gelinas J.N., Khodagholy D. “Enhancement-mode ion-based transistor as a comprehensive interface and real-time processing unit for in vivo electrophysiology.” Nat. Mater. vol. 19, no. 6., pp 679–686, Mar. 2020. ↩︎
- Lee H., Lee S., Lee W., Yokota T., Fukuda K., Someya T. “Ultrathin Organic Electrochemical Transistor with Nonvolatile and Thin Gel Electrolyte for Long-Term Electrophysiological Monitoring” Funct. Mater., vol. 29, no. 48, Sep. 2019. ↩︎
- Savva A., Wustoni S., Inal S., “Ionic-to-electronic coupling efficiency in PEDOT:PSS films operated in aqueous electrolytes” J. Mater. Chem. C, vol. 6, no. 44, pp 12023-12030, Jul. 2018. ↩︎
- M. V. Voinova, M. Rodahl, M. Jonson, B. Kasemo, “Viscoelastic Acoustic Response of Layered Polymer Films at Fluid-Solid Interfaces: Continuum Mechanics Approach” Phys. Scr., vol. 59, no. 5, pp 391, 1999. ↩︎