Electrospinning is a powerful and versatile technique used to produce nonwoven ultrafine fibers with controlled microstructures. Electrospinning can be used to fabricate fibers of different diameters ranging from 20 nm to more than 10 µm. Fibers can be fabricated from solution, emulsion, suspension, sol-gel or slurry composed from different materials like polymers (natural, semi-synthetic and synthetic), ceramics, metals and/or different types of additives. Its ability to process a wide range of materials and produce fibers with tailored properties has made it a cornerstone in applications like textiles, medical & healthcare, energy, filtration, packaging, automotive, and aerospace.
One of the strengths of electrospinning is its adaptability in producing a variety of fibers with a range of morphologies. Here we highlight a few different types of nonwoven electrospun fiber morphologies obtained with electrospinning.
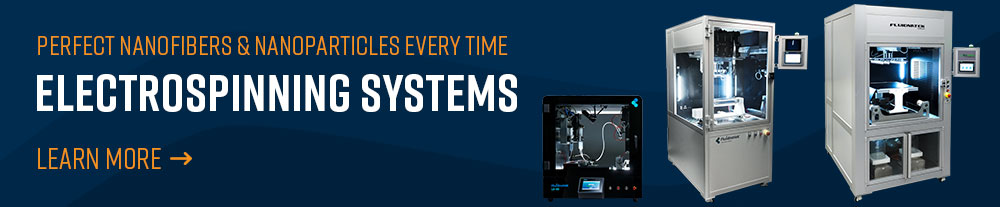
Single Phase Fibers
Single phase electrospun fibers are the most common fibers fabricated by electrospinning. These are fibers made by processing a single, or blended material in the same solution, through one needle. Depending on solution properties and processing parameters, fiber diameter can be fine-tuned from nanoscale to microscale, based on application needs. Figure 1 showcases a couple examples of fibers ranging from the nanoscale, sub-micron and microscale made with the Fluidnatek equipment.
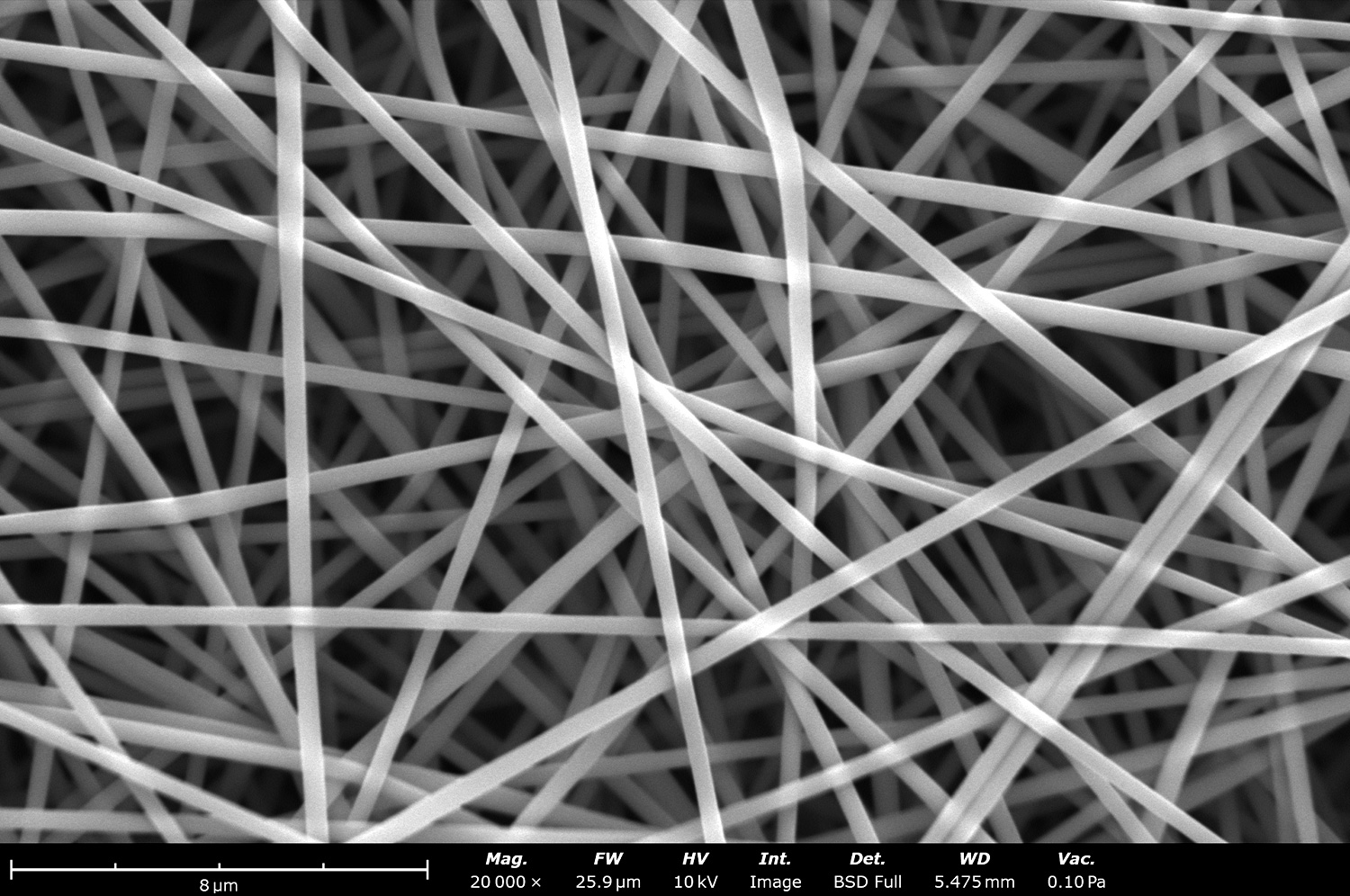
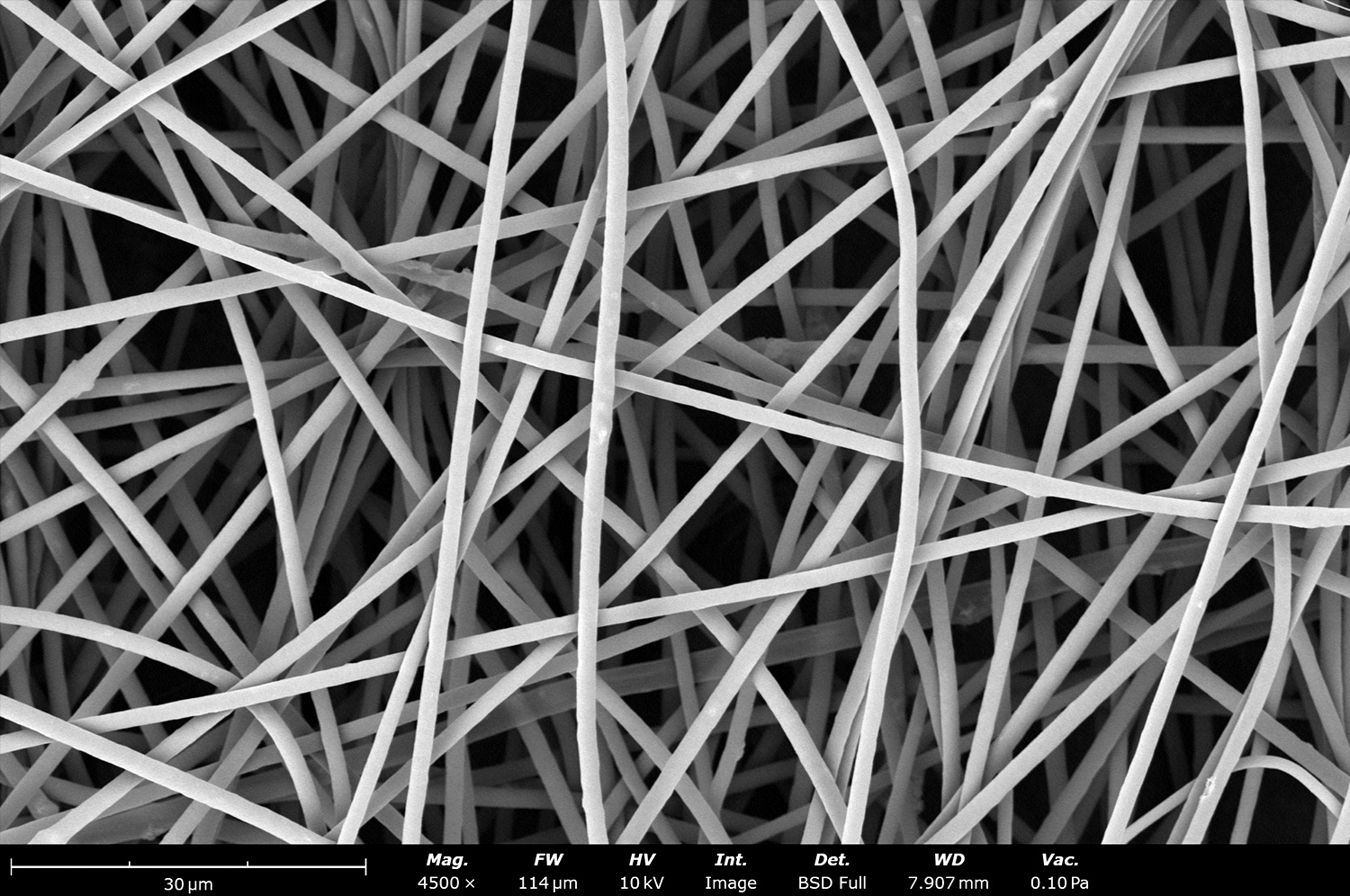
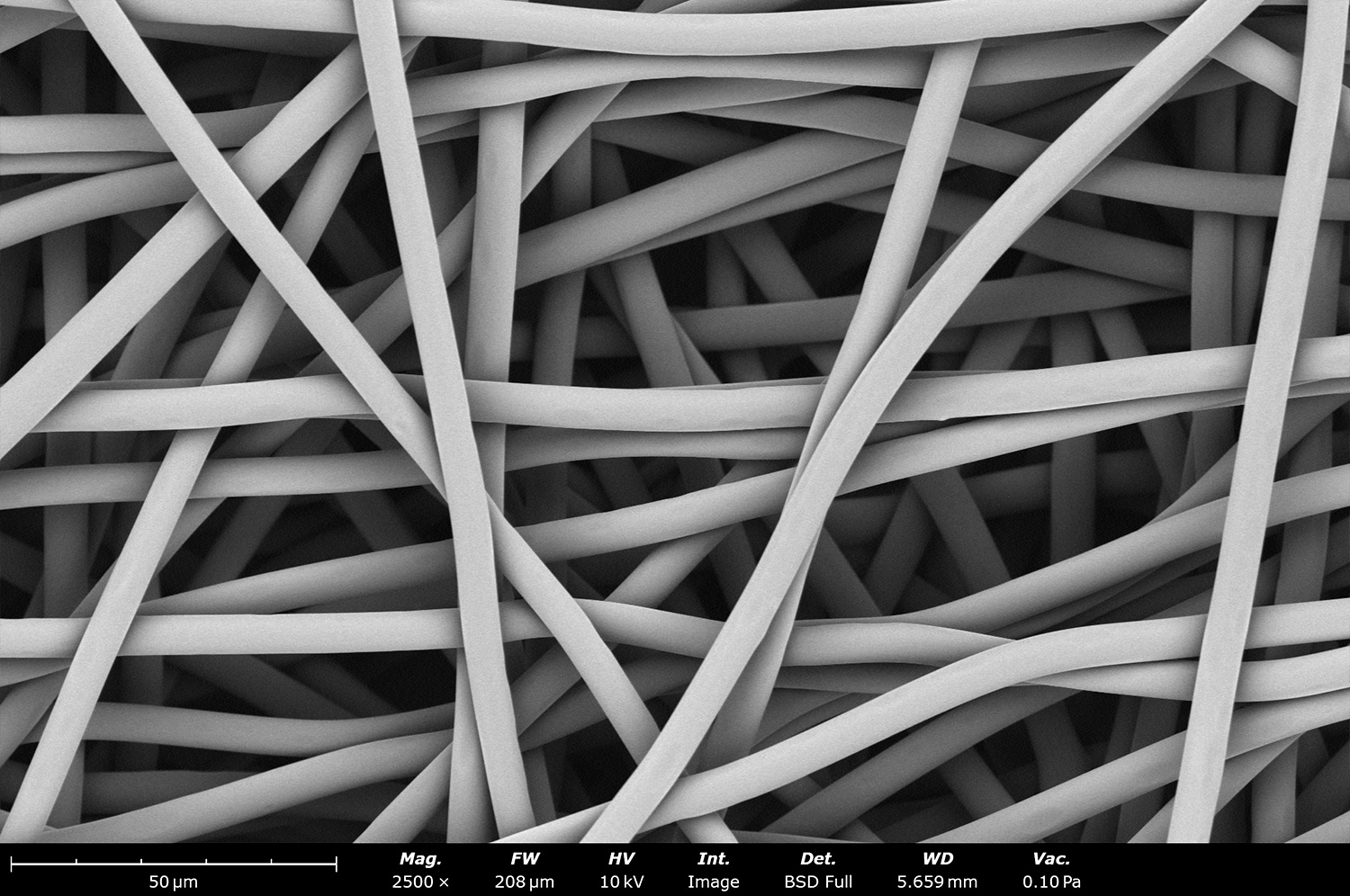
Fibers Embedded with Particles
Electrospinning offers the innate capability of processing solutions that contain particles, or other additives. If the particle or additive of interest is well mixed, suspended, and homogeneous, fibers with well dispersed particles can be fabricated (Figure 2). Researchers at Lawrence Livermore National Laboratory fabricated fibers composed of polyvinylpyrrolidone (PVP) and gallium nitrate which were then thermally treated to generate gallium oxide nanofibers.1 These fibers can be used as integrated sensors for energy systems.
Hashem et. al., fabricated bactericides fibers by loading polyvinyl chloride (PVC) with different metal-organic frameworks (MOFs) to increase antibacterial activity.2 These types of antibacterial fibers are used in applications like food packaging, and medical devices.
Beaded Fibers
Electrospun beaded fibers, also known as bead-on-string, are not usually desired. They are characterized by beads in micron sizes connected to fibers in the nanoscale. However, for certain applications such as drug delivery and filtration, these structures could prove to be beneficial. By modulating the solution concentration and charge density or processing parameters like relative humidity, needle-to-collector distance, flow rate and voltage, beaded fibers can be fabricated.
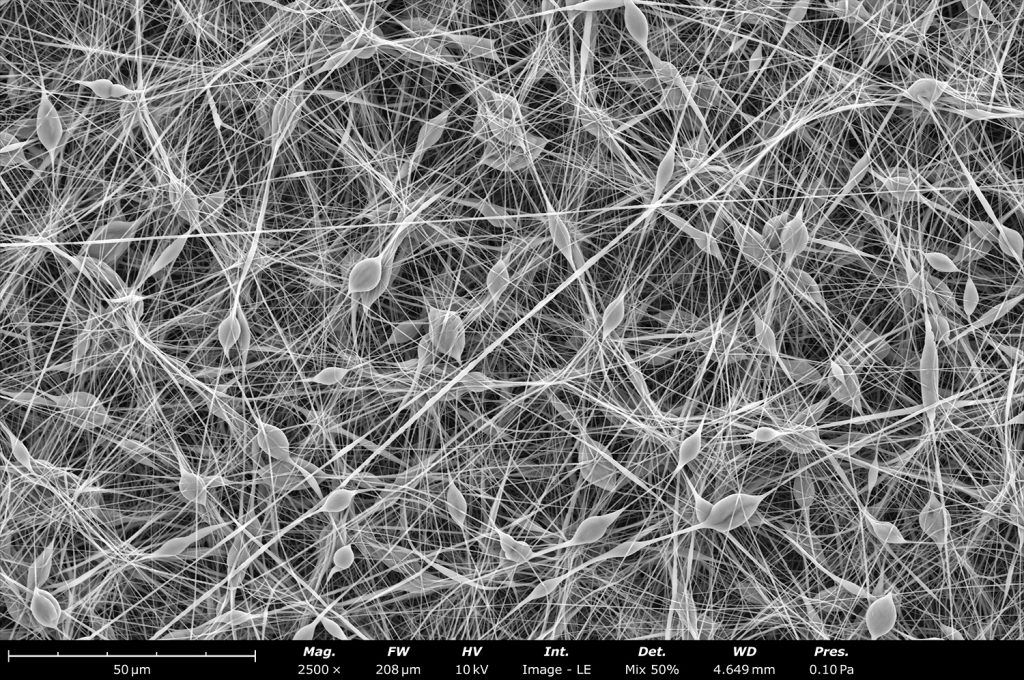
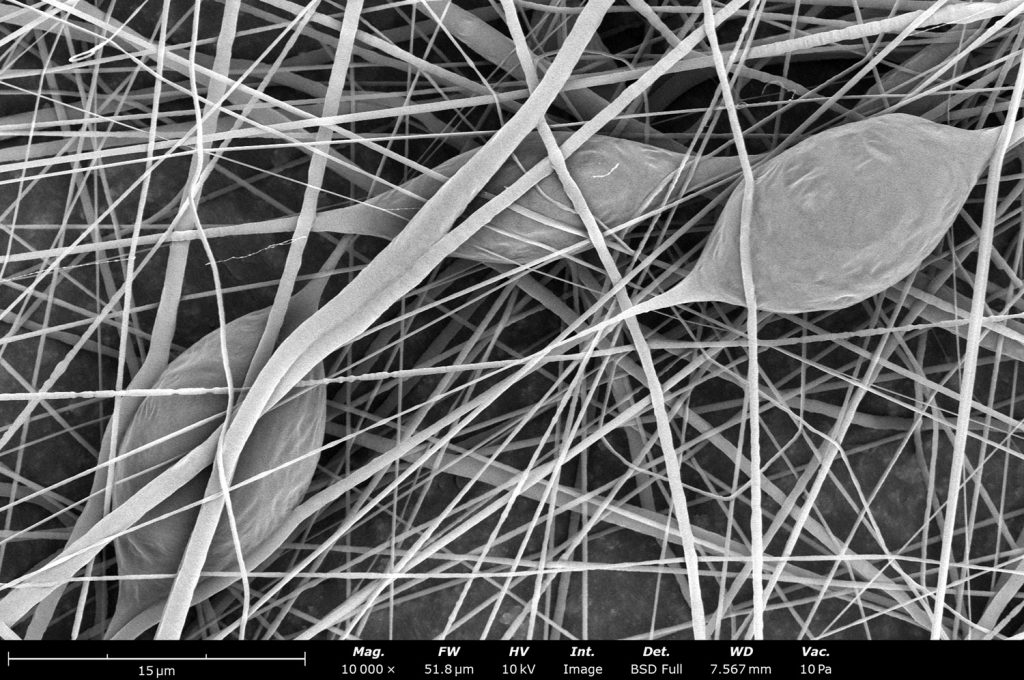
In drug delivery applications, beaded fibers could prove to beneficial since they can encapsulate larger quantities of drugs while maintaining the integrity of the fibers. The bead structure also prevents burst drug release when compared to single-phase fibers (Figure 3). Bead-on-string structure can also improve filtration efficiency as it prevents high pressure resistance. Thanks to the microstructure tunability, beads can be generated with different sizes, shapes and even include nanoscale porosity, which will benefit air flow and adsorption of particles and other media.3
Fibers with Surface Porosity
In certain applications such as catalysis, filtration, tissue engineering and drug delivery, fibers with a porous surface can be advantageous. Since a higher surface area is achieved with these types of fibers, it is expected that smaller quantities (ex. thickness and area density) will be needed to achieve the targeted function. In tissue engineering porous surfaces can help cells anchor during proliferation and in drug delivery it can assist delivery of active pharmaceutical ingredients (API).
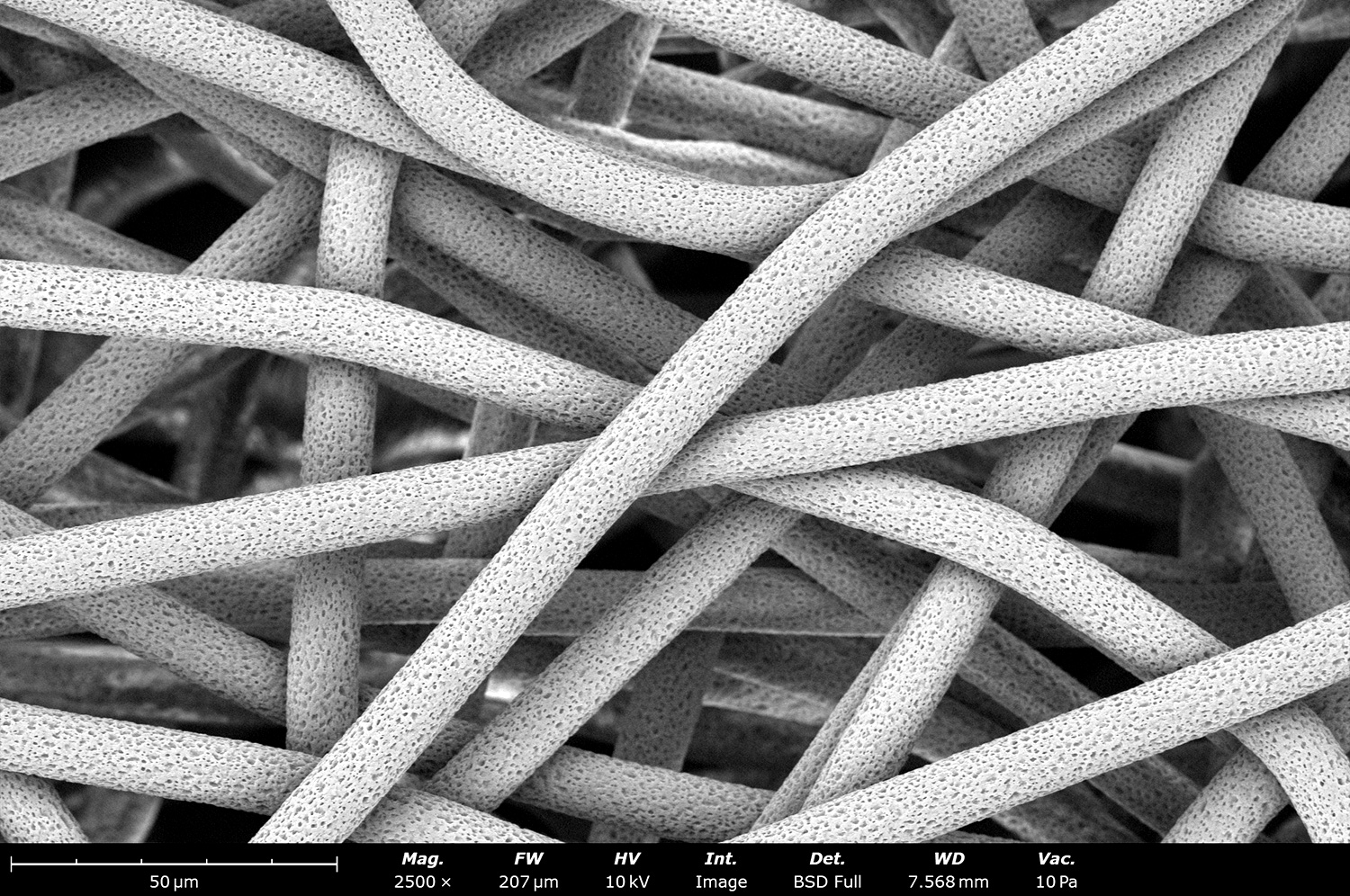
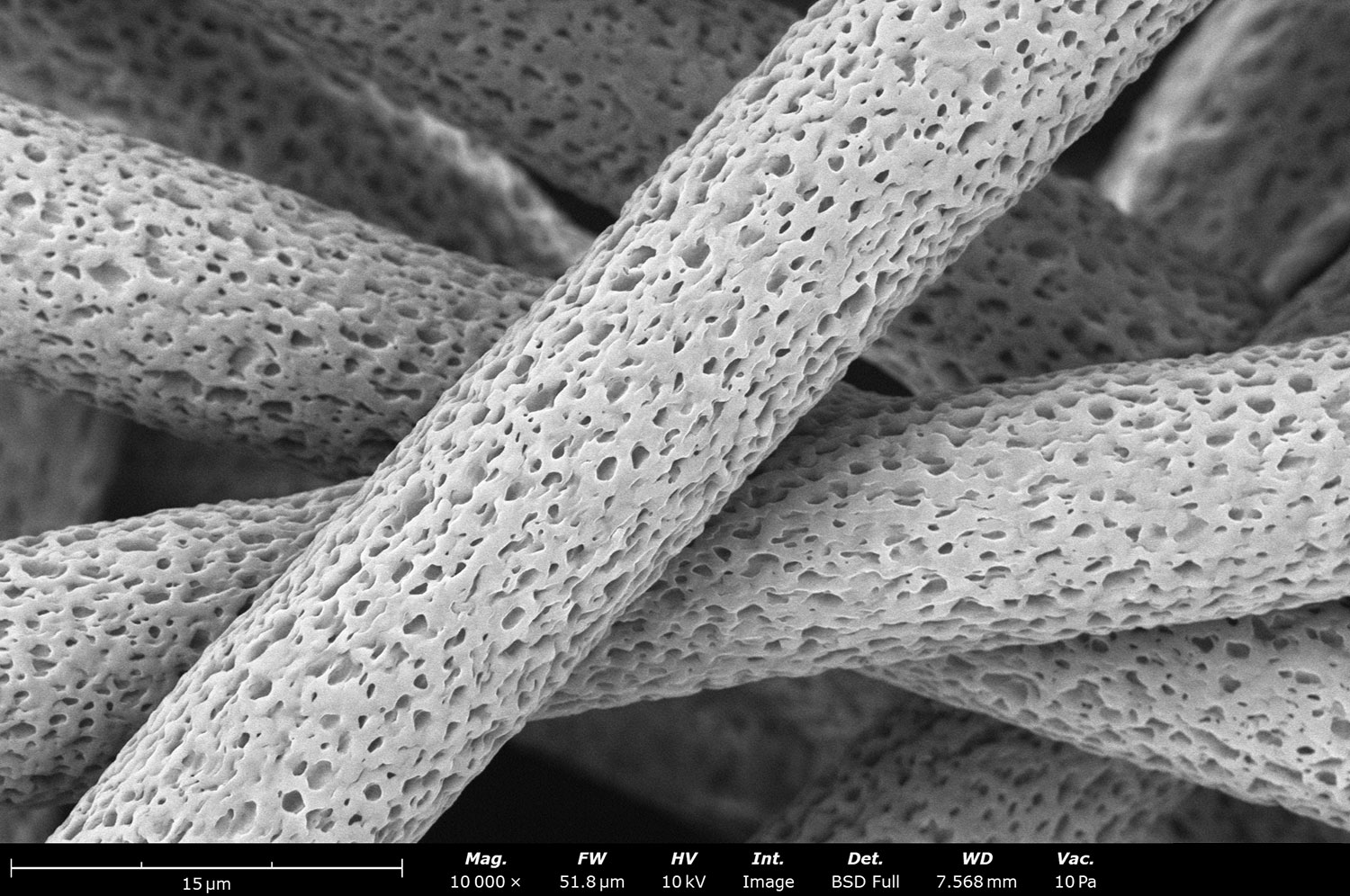
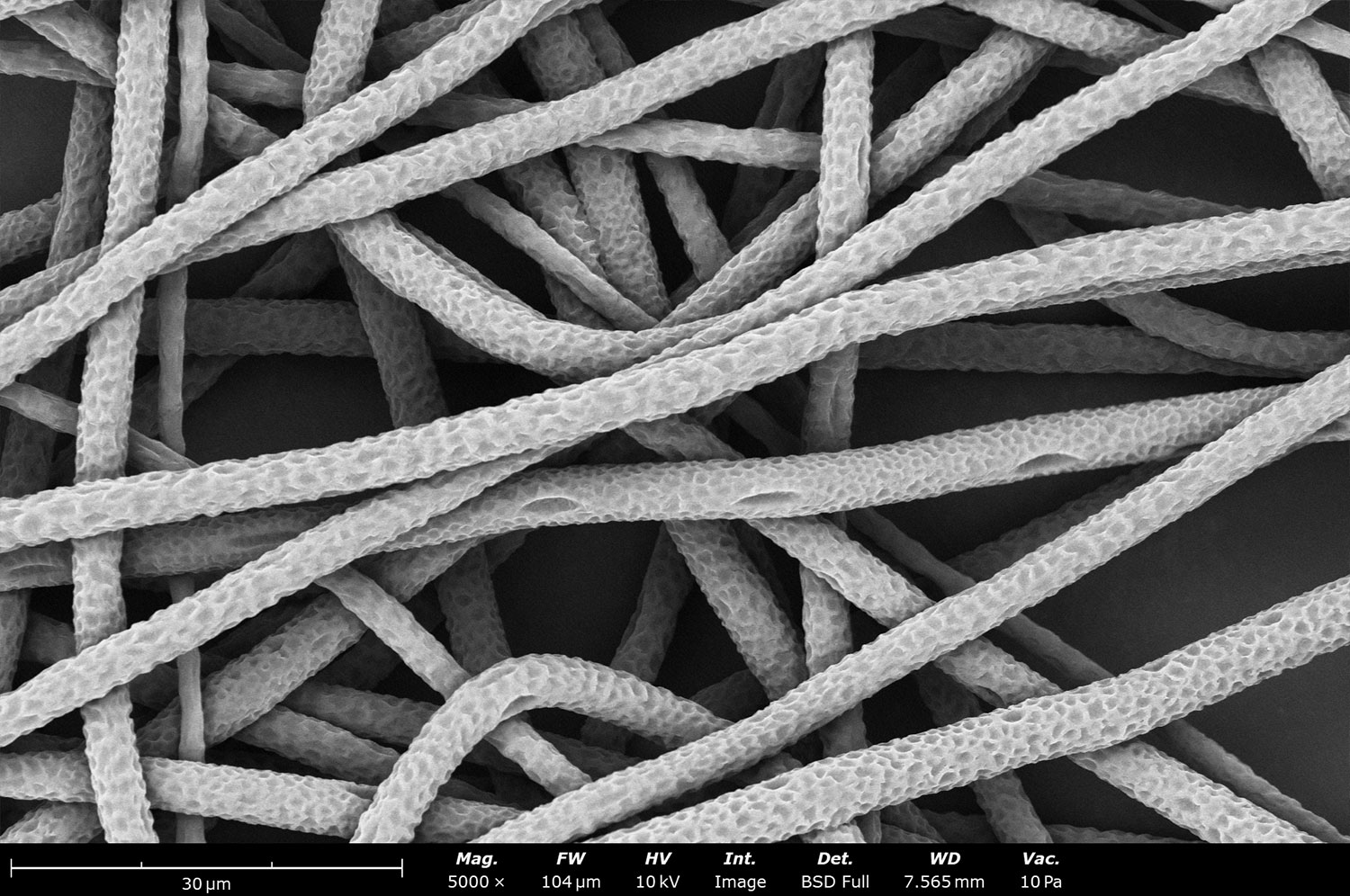
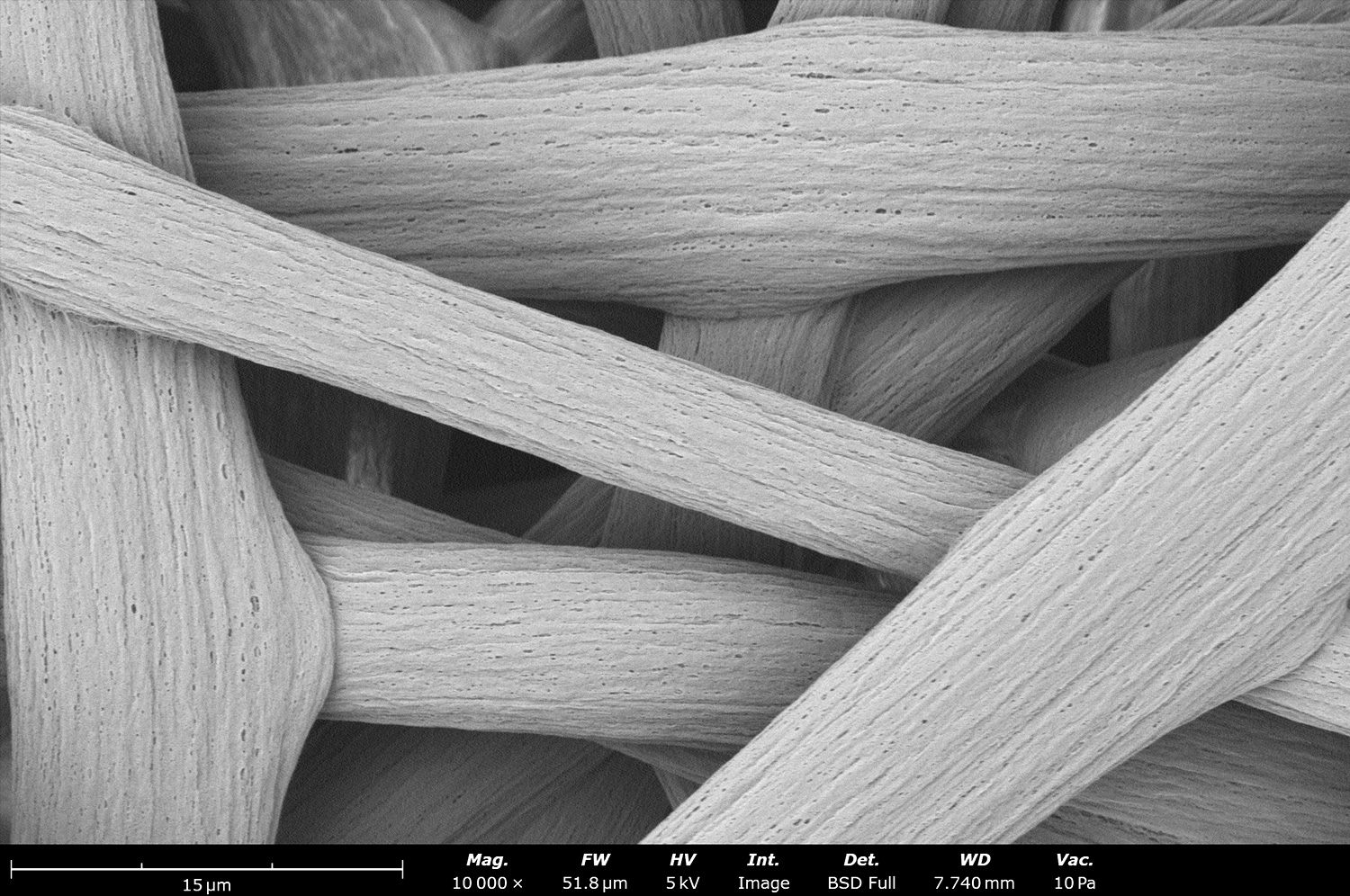
Formation of surface pores is known as mesopore formation. There are various ways to generate fibers with surface porosity.4 The most common method is to use a solvent with high vapor pressure that can evaporate quickly during fiber formation resulting in fibers like the one shown on Figure 4a and 4b for PCL using dichloromethane as the solvent. Another method is to electrospin hydrophobic polymers at relatively high humidities. As the solvent evaporates, cool down effect results in water droplets collecting on the surface of the fiber resulting in porous structures. Figure 4c shows this example with the microstructure of PCL using a solvent mixture of chloroform and methanol with the process at 70% relative humidity.
An alternative method to induce mesopore formation is by post-processing the sample. Fibers are generated using a blend of two different polymers and then one of them is selectively removed. Figure 4d shows an example where a blend of PCL:PET fibers were initially fabricated and PCL was selectively removed by exposure to acetone. Similarly, salts from a blend of PCL:PET:HEPES-salt generated fibers, can be leached out by water to generate a porous fibers.5
Mesopore formation followed by micropore formation can further increase the surface area by post-processing the sample. For example, researchers at Los Alamos National Laboratory developed electrospun PAN fibers containing SiO2. These PAN-SiO2 fibers were carbonized and SiO2 was dissolved to induce mesopore formation. Further post-processing of these carbonized nanofibers with sodium hydroxide and thermally treated under inert conditions induced the formation of micropores. This resulted in a further increase in fiber pore volume while maintaining fiber integrity, resulting in excellent capacitive deionization for desalination applications.6
Multiaxial Fibers
These are electrospun fibers generated when two, or more, solutions are processed with a needle contained within another needle. Figure 5a showcases the typical single-phase fibers along with two types of multi-axial arrangements (coaxial and triaxial). When compared to single phase, multiaxial fibers can encapsulate one material within another. This offers advantages like encapsulating additives protecting it from possible harsh environments. Applications like drug delivery can benefit from multiaxial fibers, where the drug release rate can be controlled based on core and shell thickness, along with affinity between drug and material. This process can also be used to fabricate hollow fibers. Figure 5b and 5c show case electrospun PLA hollow fibers fabricated with the Fluidnatek technology.
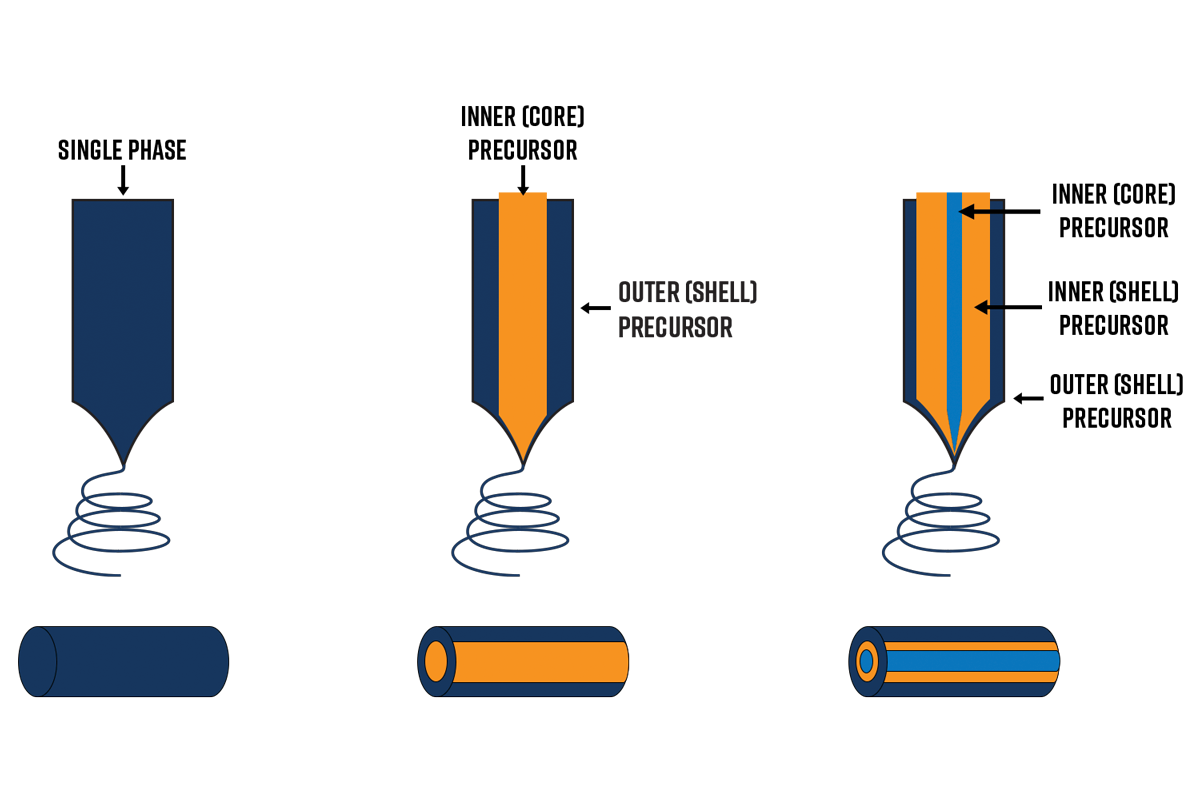
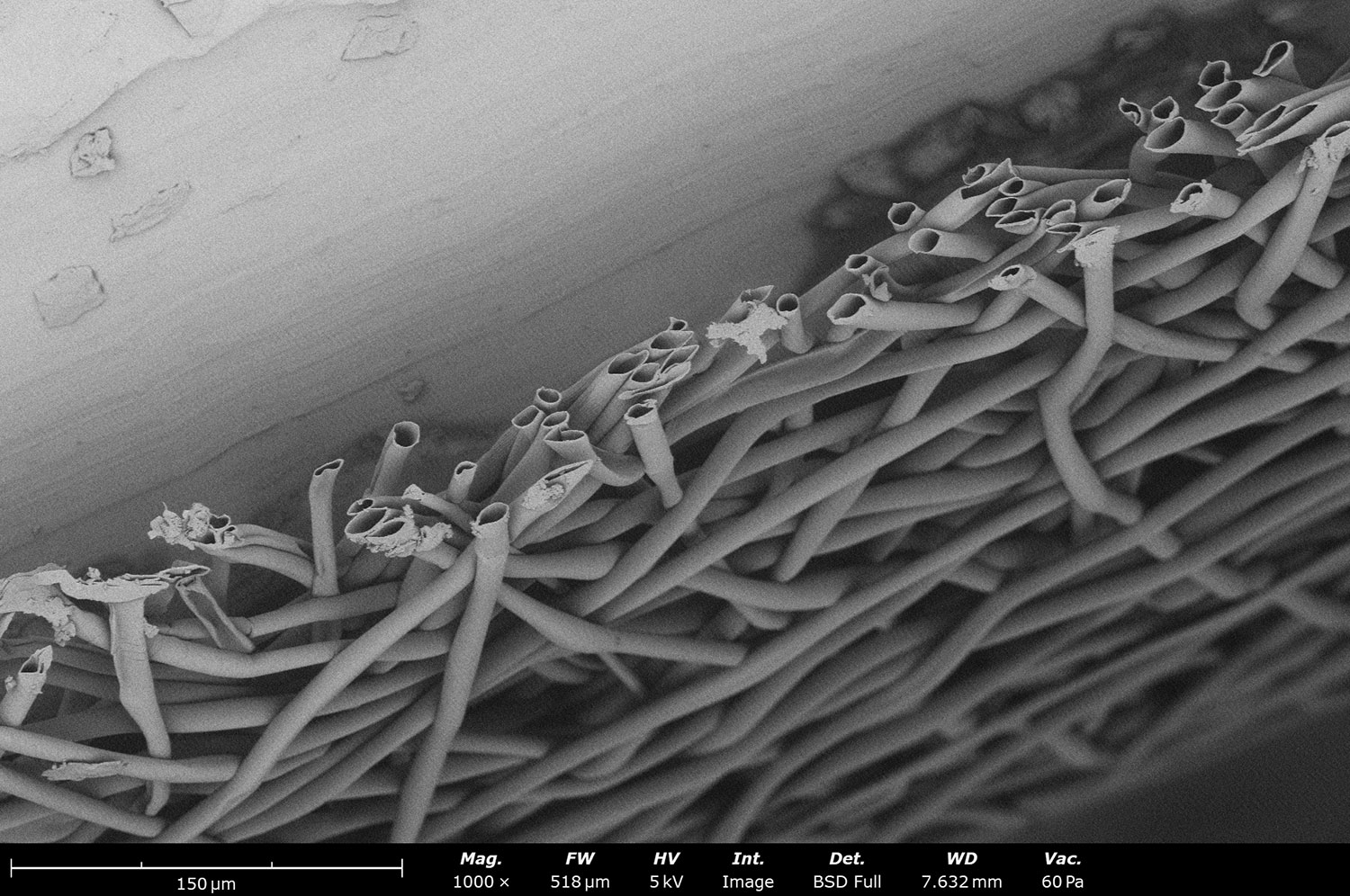
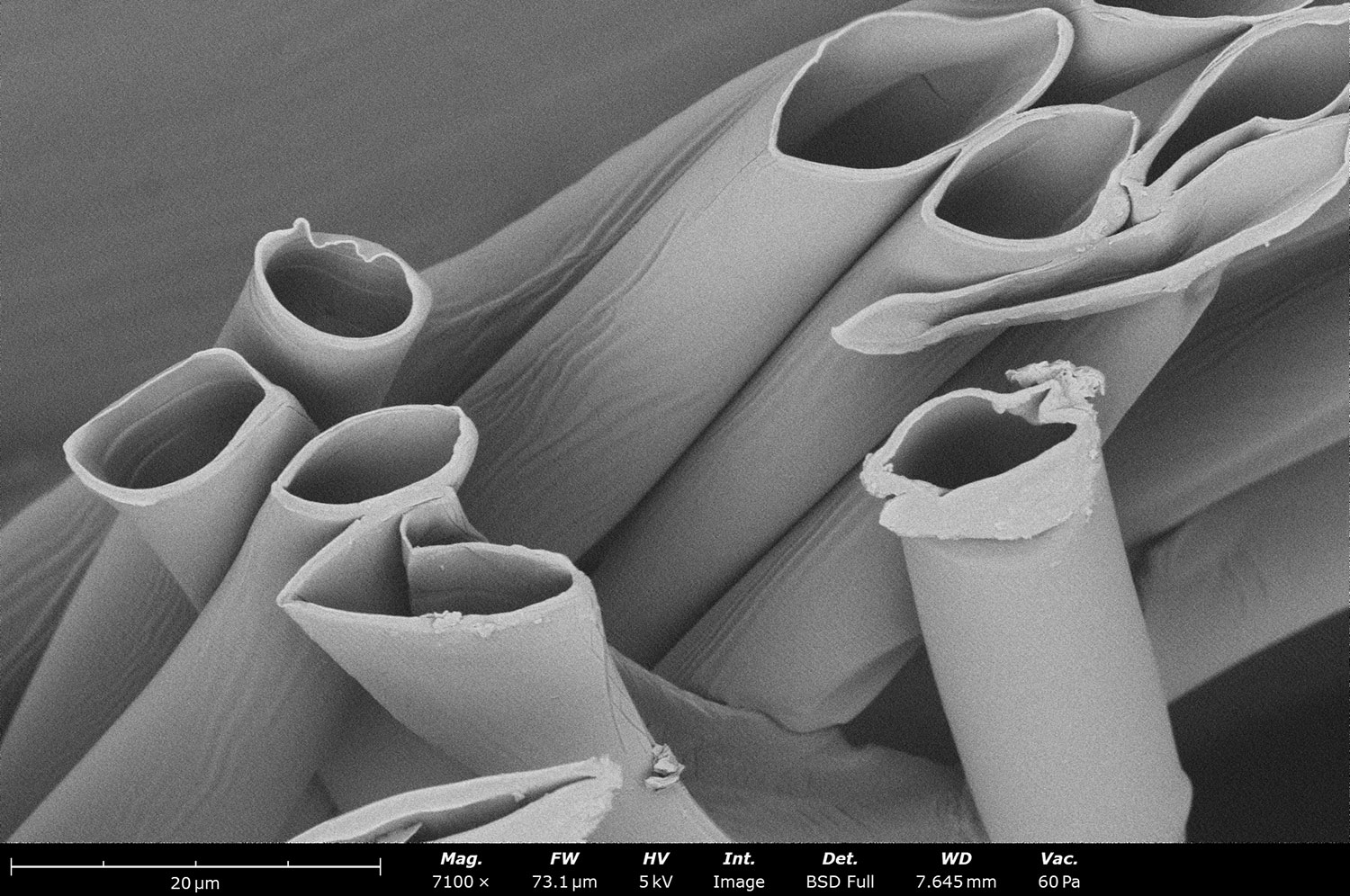
Conclusions
Electrospinning stands as a versatile and innovative technique for fabricating diverse fiber microstructures. Its adaptability in controlling fiber diameter, alignment, and composition has made it invaluable across a broad spectrum of applications like medical devices, drug delivery, energy storage and filtration. The potential of electrospinning is vast, making it a key tool for researchers and industries aiming to push the boundaries of fiber fabrication technologies. As advancements continue, electrospinning is set to play a pivotal role in shaping the future of materials science and engineering.
References
- Makeswaran, N.; Kelly, J. P.; Haslam, J. J.; McKeown, J. T.; Ross, M. S.; Ramana, C. V. Crystallization, phase stability, microstructure, and chemical bonding in GA2O3 nanofibers made by electrospinning. ACS Omega 2022, 7 (36), 32816–32826. https://doi.org/10.1021/acsomega.2c05168 ↩︎
- Hashem, M. H.; Wehbe, M.; Damacet, P.; Habbal, R. K. E.; Ghaddar, N.; Ghali, K.; Ahmad, M. N.; Karam, P.; Hmadeh, M. Electrospun Metal–Organic Framework-Fabric nanocomposites as efficient bactericides. Langmuir 2023, 39 (27), 9503–9513. https://doi.org/10.1021/acs.langmuir.3c01039 ↩︎
- Zhao, H.; Chi, H. Electrospun Bead-on-String fibers: Useless or something of value? In InTech eBooks; 2018. http://dx.doi.org/10.5772/intechopen.74661 ↩︎
- Cao, X.; Chen, W.; Zhao, P.; Yang, Y.; Yu, D.-G. Electrospun porous nanofibers: Pore−Forming mechanisms and applications for photocatalytic degradation of organic pollutants in wastewater. Polymers 2022, 14 (19), 3990. https://doi.org/10.3390/polym14193990 ↩︎
- Nanoscale porosity on polymeric sintered electrospun capsules for long-term controlled release. American Chemical Society. https://acs.digitellinc.com/p/s/nanoscale-porosity-on-polymeric-sintered-electrospun-capsules-for-long-term-controlled-release-611926 ↩︎
- Waugh, J. B.; Babu, S. K.; Kang, Q.; Moehring, N. K.; Benavidez, A.; Wang, X.; Kidambi, P. R.; Pintauro, P. N.; Spendelow, J. S. Hierarchically porous electrospun carbon nanofiber for high-rate capacitive deionization electrodes. Desalination 2024, 584, 117610. https://doi.org/10.1016/j.desal.2024.117610 ↩︎