Wound care solutions are essential in medicine to protect injuries from infection, manage exudate, and create an optimal healing environment. Effective solutions promote faster and more efficient healing, reducing the risk of complications and scarring. There is a continuous quest for innovative wound care solutions to improve patient outcomes and overall quality of life by supporting tissue regeneration and providing pain relief. Electrospinning is an advanced technology that has demonstrated the potential to transform wound care through its ability to create nanofibrous scaffolds with tailored properties. In this blog, we’ll delve into the applications of electrospinning in wound healing and explore how this technique is helping shape the future of healthcare.

Electrospinning
Electrospinning is a versatile technique used to fabricate polymeric nanofibers, ranging from a few nanometers to micrometers, by applying an electric field to draw fibers from a polymer solution. The electrospinning process can achieve precise control over the size, shape, and mechanical properties of the fibers enabling the customization of dressings to specific wound types and healing stages.
Nano- or micro-fibrous mats with high surface area and porosity can be fabricated to mimic the extracellular matrix (ECM) structure, providing an ideal environment for cell attachment, proliferation, and tissue regeneration, making them perfect for wound healing applications.1 Furthermore, the fibers can be tuned for distinct bioresorbability profiles (e.g. via hydrolysis) such that successful integration of native tissues is simultaneously promoted. Additionally, their versatility in accommodating a wide range of materials—including natural and synthetic polymers, and bioactive substances—enables the fabrication of dressings with antimicrobial properties and controlled drug release.2
Applications in Wound Healing
Drug-enhanced Dressings:
Electrospun nanofibers can serve as effective carriers for delivering therapeutic agents directly to the wound site. By encapsulating drugs within the fibers, controlled release kinetics can be achieved, ensuring sustained drug release over an extended period. The gradual release of the drug from the nanofibrous dressing reduces the risk of infection and accelerates tissue regeneration.2 This targeted approach minimizes systemic side effects and enhances the therapeutic efficacy of drugs, such as antimicrobials, growth factors, and anti-inflammatory agents.
Tissue Engineering Scaffolds:
Electrospun nanofibrous scaffolds closely resemble the native ECM architecture, making them ideal substrates for tissue regeneration. The fibrous structures serve as support for the growth of new tissue, guiding the repair process while maintaining the structural integrity of the wound area.3 More specifically, they provide a three-dimensional framework for cell adhesion, migration, and proliferation, facilitating the formation of new tissue. By incorporating bioactive molecules and promoting cell-specific signaling, electrospun scaffolds can accelerate wound closure and promote the regeneration of functional tissue. The area of skin regeneration and, tangentially, skin substitutes are impacted by this. For example, by incorporating cells into the electrospun fiber scaffolds, researchers have developed living tissue constructs that can be used for skin regeneration, offering hope for patients with severe burns or chronic wounds.1 Commercially available examples of this type of product include RESTRATA® MiniMatrix™ (Acera Surgical), RESTRATA® Meshed™ (Acera Surgical), and NanoWhiskers™ (Nanofiber Solutions). Figure 1 provides an example of micronized nanofibers for wound healing, similar to the MiniMatrix™ or NanoWhiskers™, which can be injected directly to wound sites or applied to tunneling or complex-shaped wound geometries.
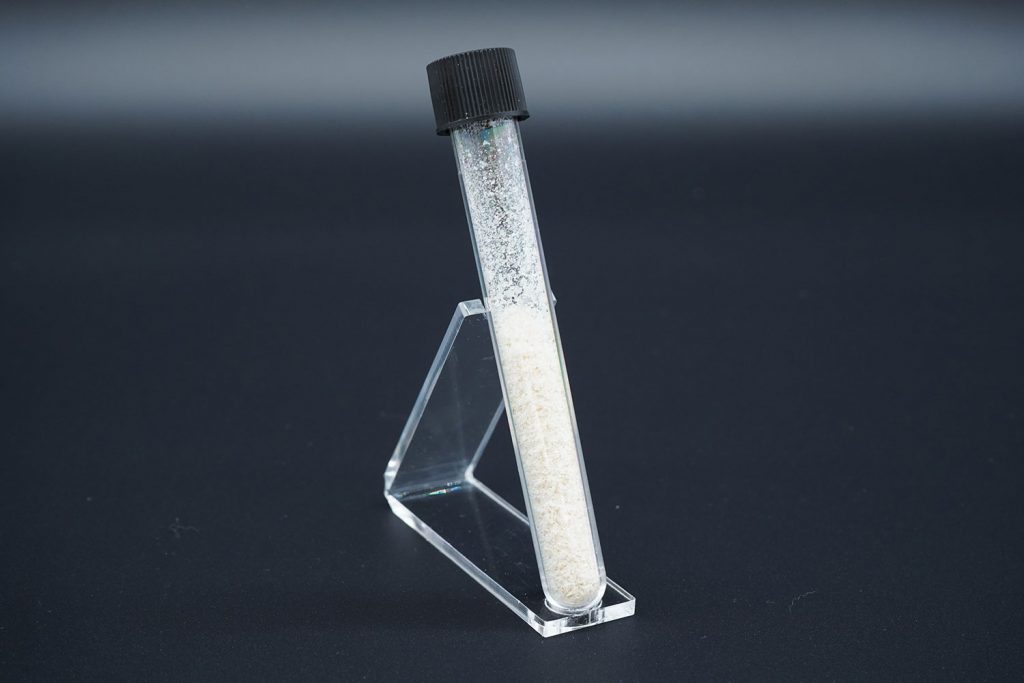
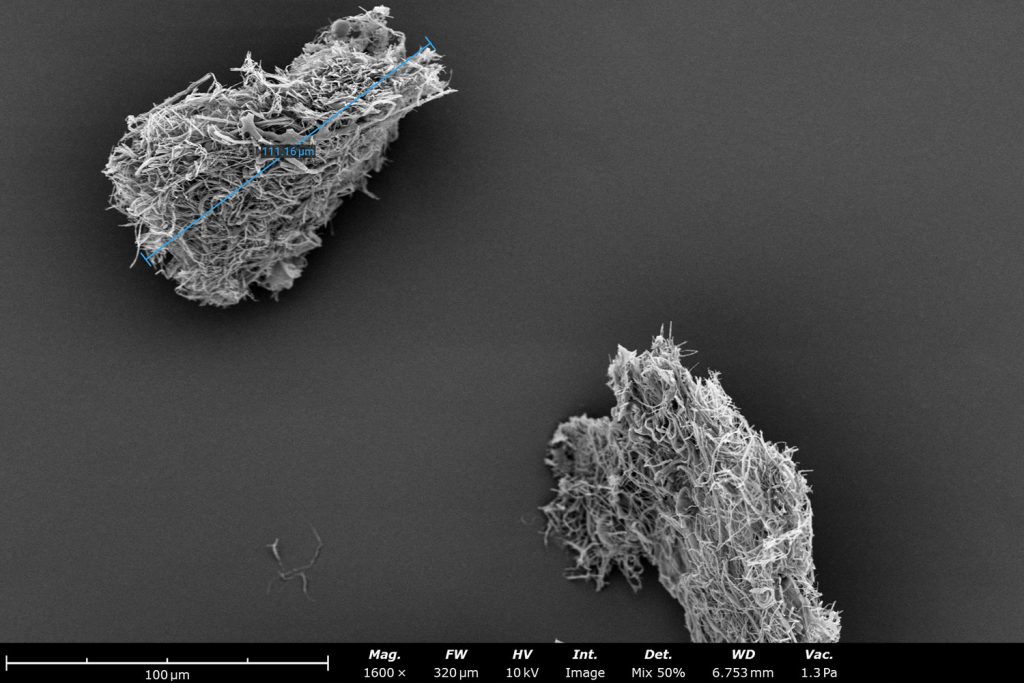
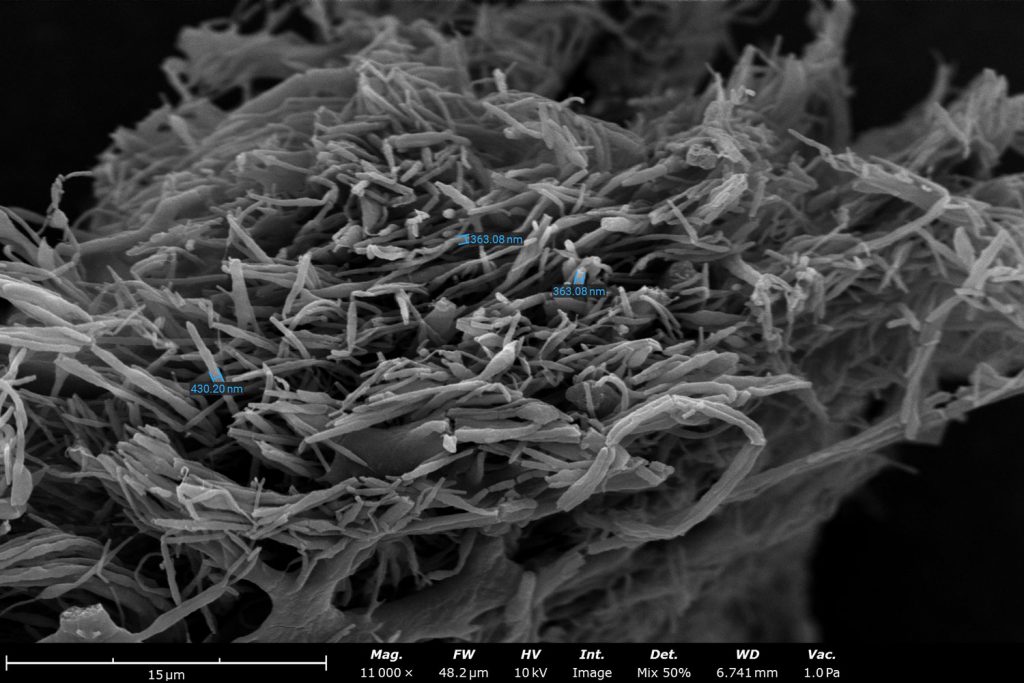
Skin Substitutes:
Electrospun materials can act as temporary or permanent skin substitutes, providing protection and stimulating the body’s natural healing processes.4 These fibrous meshes can be formed from many different materials, including both natural and synthetic sources, which allows for tailoring of the mesh to meet specific needs like mechanical strength and biocompatibility (Figure 2). Additionally, ethical or religious objections of patients can be avoided with these types of materials. FDA-cleared commercially available examples of this kind of material are the RESTRATA® Sheet™ (Acera Surgical), Phoenix™ Wound Matrix® (RenovoDerm), and Anthem™ Wound Matrix (RenovoDerm), applicable for both acute and chronic wound treatment.
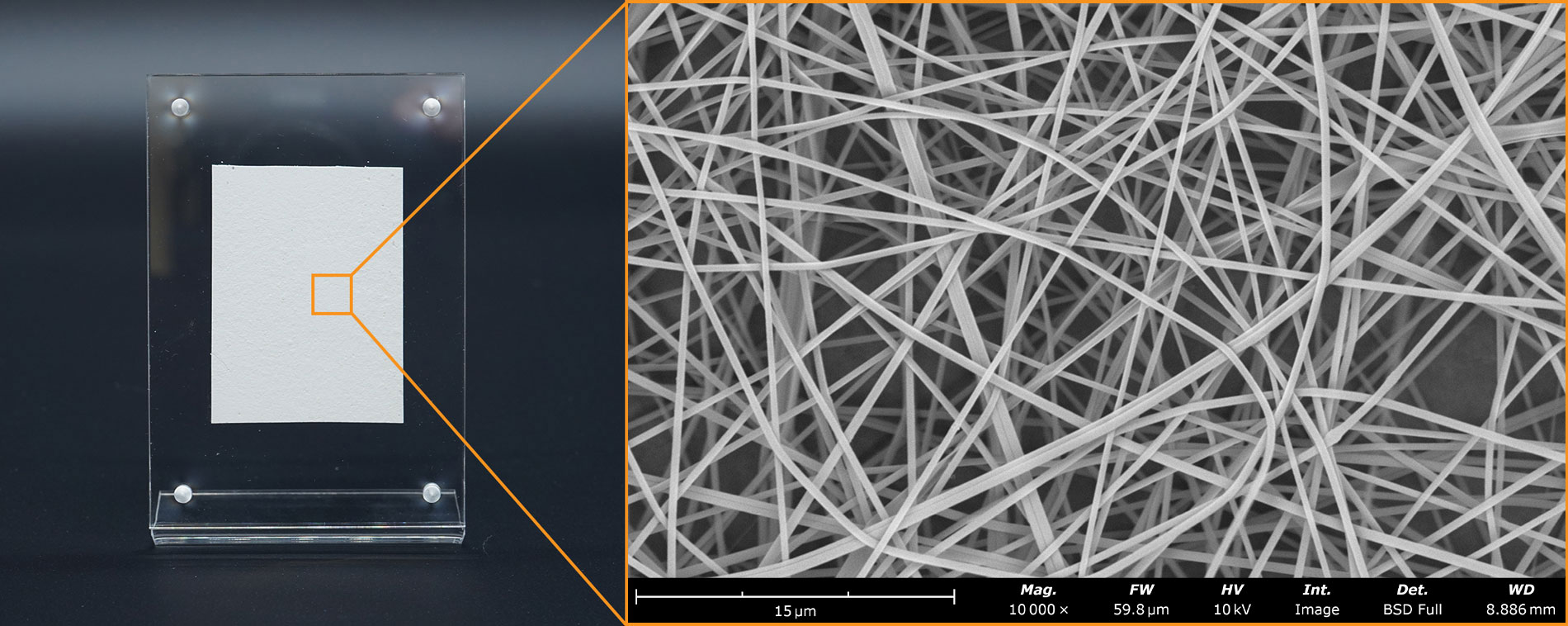
Antimicrobial Dressings:
Wound infections pose a significant challenge in healthcare, leading to delayed healing and potential complications. Electrospun nanofibers can be functionalized with antimicrobial agents, such as silver nanoparticles, antibiotics, or antimicrobial peptides, to create dressings with intrinsic antimicrobial properties.
These antimicrobial dressings prevent bacterial colonization, reduce the risk of infection, and create a conducive environment for wound healing. For example, ginger essential oil combined with hydrolyzed chitosan nanofiber membranes have shown great antibacterial and biocompatibility for treatment of wound infections.5 Similar natural polymer nanofiber dressings, as seen in Figure 3, can be modified to contain these helpful additives.
Hemostatic Agents:
Electrospun nanofibers can be engineered to possess hemostatic properties, making them effective hemostatic agents for controlling bleeding in traumatic injuries or surgical procedures. By promoting platelet adhesion and aggregation, these nanofibers facilitate the formation of blood clots, thereby reducing blood loss and promoting wound stabilization. A couple of the ways these electrospun meshes can be tailored to promote clotting is through specific materials, additives, or intentional porosity control.6, For example, materials with natural clotting properties like chitosan, can be paired with additives like tannic acid and zinc-based metal organic frameworks (MOFs), to have a positive synergistic effect on hemostatic performance.
Future Directions
The field of electrospinning continues to evolve, with ongoing research focusing on refining fabrication techniques, optimizing material properties, and exploring new applications in wound healing. Future advancements may involve the development of smart scaffolds capable of responding to specific cues within the wound microenvironment, personalized medicine approaches tailored to individual patient needs, and integration with emerging technologies such as 3D bioprinting and bioinks.
Conclusion
Electrospinning holds immense potential for advancing wound care by offering tailored solutions for promoting healing, combating infections, and improving patient outcomes. Through its versatility and ability to engineer nanofibrous materials with precise properties, electrospinning is poised to reshape the landscape of wound healing, offering hope for more efficient, cost-effective, and patient-centric approaches to healthcare. As research and commercialization in this field progresses, we can anticipate witnessing transformative innovations that translate into tangible benefits for patients worldwide.
References
- Z. Hu et al., “Cell electrospinning and its application in wound healing: principles, techniques and prospects,” Burns and Trauma, vol. 11, Jan. 2023. ↩︎
- A. Akhmetova and A. Heinz, “Electrospinning proteins for wound healing purposes: Opportunities and challenges,” Pharmaceutics, vol. 13, no. 1, p. 4, Dec. 2020. ↩︎
- S. Guo, P. Wang, P. Song, and N. Li, “Electrospinning of botanicals for skin wound healing,” Frontiers in Bioengineering and Biotechnology, vol. 10, Sep. 2022 ↩︎
- R. Mohamadinooripoor, S. Kashanian, and E. Arkan, “An overview on wound dressings and sutures fabricated by electrospinning,” Biotechnology and Bioprocess Engineering, vol. 28, no. 1, pp. 17–35, Feb. 2023. ↩︎
- M. Berechet et. al., “An antimicrobial Peptide-Loaded Antioxidant and Antimicrobial Properties of Hydrolysed Collagen Nanofibers Loaded with Ginger Essential Oil, Materials 2023, vol. 16, no 4, pp. 1438. ↩︎
- A. McCarthy et. al., “Large-scale synthesis of compressible and re-expandable three-dimensional nanofiber matrices” Nanoselect, vol. 97, Feb 2021. ↩︎