Quartz Crystal Microbalance with Dissipation Monitoring (QCM-D) is a versatile analytical technique that can provide real-time, quantitative data on mass changes and viscoelastic properties of thin films and surfaces, making it an essential tool in many scientific and industrial fields. Some of the key applications of QCM-D include:
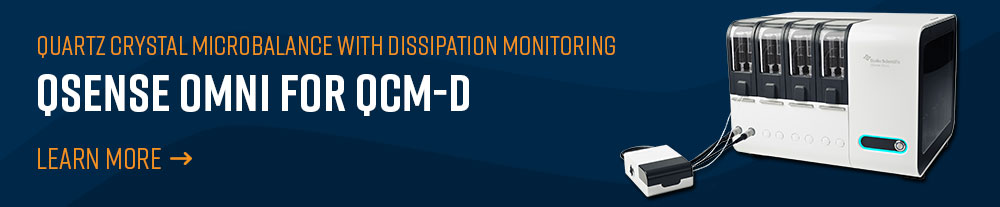
Biomolecular Interactions
QCM-D is highly sensitive to mass changes on a surface, making it ideal for studying how molecules, such as proteins, lipids, or polymers, adsorb onto surfaces.1 This is important in biomaterials research, sensor development, and surface chemistry.
When a molecule binds onto the surface of a QCM-D sensor, it changes the resonance frequency of the quartz crystal’s oscillation. The extent of this frequency shift correlates with the amount of mass adsorbed. Additionally, QCM-D measures energy loss, known as dissipation, providing insights into the rigidity or softness of the adsorbed layer. This application is critical for designing surface coatings, improving material compatibility, and understanding the fundamentals of molecular interactions at interfaces. One such experiment is illustrated in Figure 1, in which a multilayer functional film was created and tested for binding specificity.2
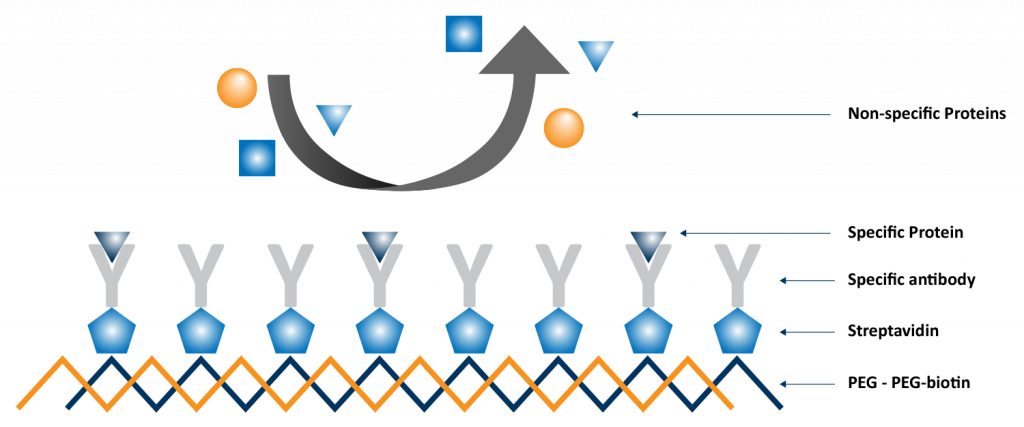
Biosensing
QCM-D can be used as a biosensor to detect specific biomolecular interactions in real-time, such as the binding of antibodies to antigens, DNA hybridization, or ligand-receptor interactions. Real-time monitoring of binding events can be critical for diagnostics and drug discovery.
For biosensing applications, the QCM-D sensor is functionalized with a recognition element (e.g., an antibody, aptamer, or receptor) that specifically binds to the target analyte.3 In the experiments shown in Figure 2, surfaces were functionalized with either protein specific antibodies or PEG specific antibodies to assess the effectiveness of lipid nanoparticle formulations for drug delivery applications. When the target binds to the sensor, it increases the mass on the sensor surface, leading to a measurable frequency shift. The ability to monitor binding events in real-time without the need for labeling the analytes is a significant advantage, making QCM-D a valuable tool for developing diagnostic devices, studying binding kinetics, and screening drugs.4
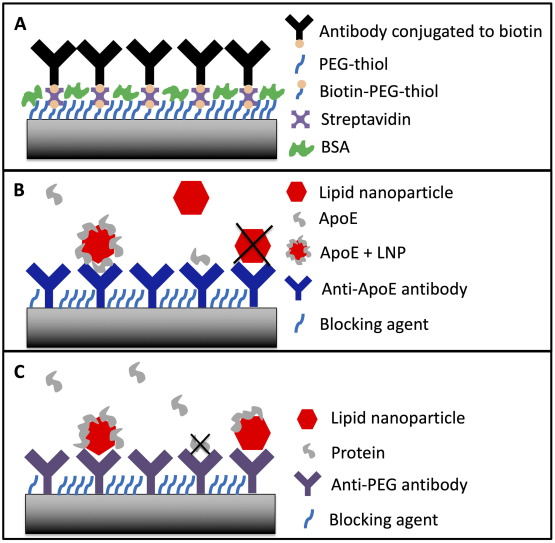
Polymer and Thin Film Characterization
QCM-D is used to analyze the deposition and behavior of thin polymer films and coatings on surfaces. It can measure changes in mass and viscoelastic properties, providing insights into film thickness, density, and mechanical properties.5
By monitoring the frequency and dissipation changes during film formation, QCM-D can determine the viscoelastic properties of the film. This includes whether the film is rigid or soft, how it changes over time (e.g., swelling, shrinking, or crosslinking), and its stability under different conditions. An example of this type of measurement is depicted in the schematic illustration in Figure 3, in which polymer chains are grafted to the QCM-D sensor surface and undergo various conformational changes. This information is crucial for applications in coatings, adhesives, and protective layers, as well as in the development of sensors and electronic devices.
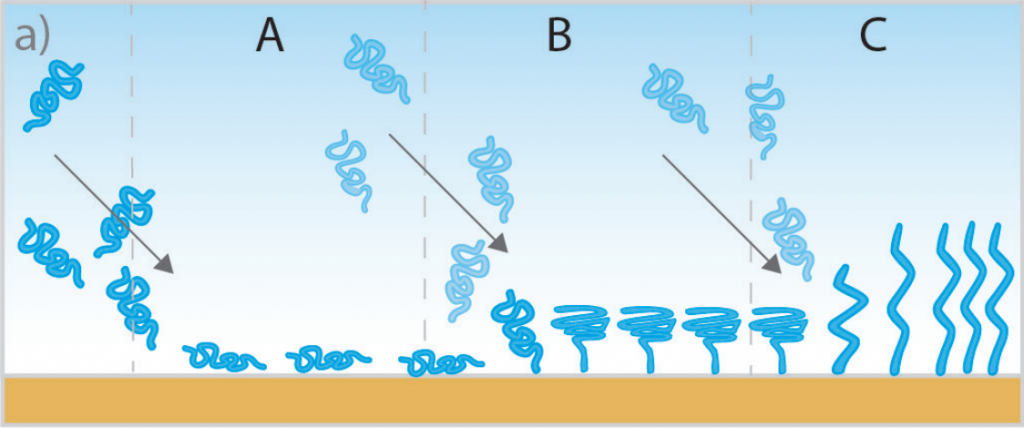
Cell Adhesion and Behavior
QCM-D allows researchers to study the dynamics of cell and biofilm adhesion, spreading, and detachment on various surfaces.6 This provides insights into cell-surface interactions and is important in tissue engineering, cell biology, and drug testing.
As cells attach and spread on the QCM-D sensor surface, they cause changes in mass and energy dissipation, which can be detected and analyzed. By observing these changes, researchers can assess how different surfaces or treatments affect cell behavior. This application is important for developing biomaterials that promote or inhibit cell adhesion, understanding wound healing processes, and screening drugs that affect cell behavior.
Membrane Studies
QCM-D is used to investigate the formation, stability, and interactions of lipid bilayers and other membrane structures.7 This is crucial in understanding cell membrane dynamics, drug delivery systems, and membrane protein interactions.
Researchers can use QCM-D to study the formation of lipid bilayers on sensor surfaces using various approaches, such as the vesicle fusion method depicted in the schematic illustration in Figure 4. QCM-D is also used to investigate the insertion of proteins into these membranes, and the interactions between membranes and various compounds (e.g., drugs, peptides, or toxins). The technique provides real-time data on the stability, fluidity, and integrity of the membrane, which is crucial for understanding cellular processes, developing drug delivery systems, and designing biosensors that mimic natural membranes.
Environmental Monitoring
QCM-D can be applied in environmental monitoring to detect and analyze pollutants, toxins, and other contaminants in air, water, or soil. It offers a sensitive method for monitoring changes in surface properties due to the adsorption of harmful substances.
By functionalizing the QCM-D sensor with materials that specifically bind to environmental contaminants, researchers can detect the presence of contaminants and pollutants with high sensitivity. For example, QCM-D can be used to monitor heavy metals, organic pollutants, PFAS, or microbial contaminants. The schematics in Figure 5 illustrate how QCM-D was used to quantify PFAS adsorption on various functionalized surfaces.8 This application is valuable for ensuring environmental safety, monitoring pollution levels, and developing methods for cleaning up contaminated sites.
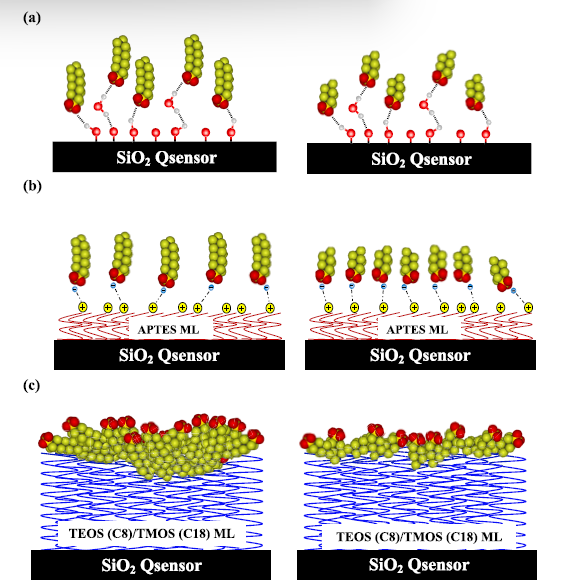
Food Science
QCM-D is used in the food and beverage industry to study the interactions of food components, such as proteins, fats, and carbohydrates, with surfaces, which is important for understanding texture, flavor release, and stability.9
By monitoring how the components in food adsorb onto surfaces, QCM-D helps in understanding factors that influence texture, flavor release, and the stability of emulsions or foams. This information can be used to optimize food formulations, improve packaging materials, and ensure the consistency and quality of food products. Additionally, QCM-D can be used to detect contaminants or spoilage agents in food, contributing to food safety and quality control. In the example illustrated in Figure 6, QCM-D was used to identify contaminated batches of wine by detecting the presence of the mycotoxin Ochratoxin A.10
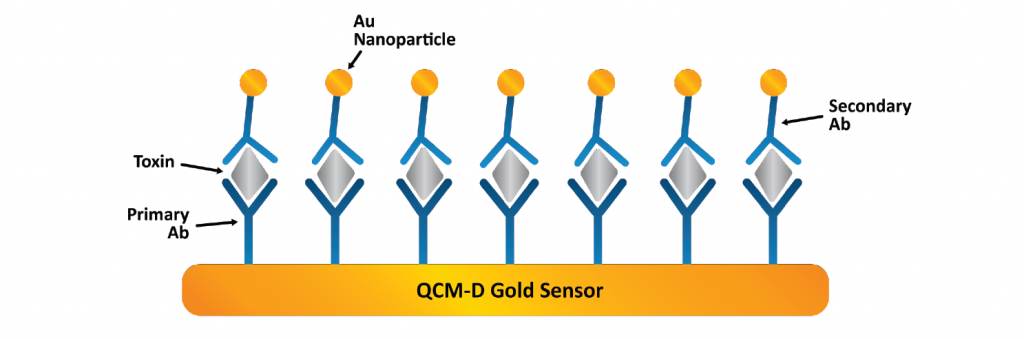
These applications demonstrate the versatility and importance of QCM-D in various fields, from fundamental research to practical industrial applications. The ability to obtain real-time, quantitative data on surface interactions makes QCM-D a powerful tool for scientists and engineers.
References
- Gong, Ningqiang, et al. “Small-molecule-mediated control of the anti-tumour activity and off-tumour toxicity of a supramolecular bispecific T cell engager.” Nature Biomedical Engineering, vol. 8, no. 5, 20 Feb. 2024, pp. 513–528, https://doi.org/10.1038/s41551-023-01147-6. ↩︎
- Kruk, Tomasz, et al. “Control of specific/nonspecific protein adsorption: Functionalization of Polyelectrolyte Multilayer Films as a potential coating for biosensors.” Materials, vol. 14, no. 24, 11 Dec. 2021, p. 7629, https://doi.org/10.3390/ma14247629. ↩︎
- Suthar, Jugal, et al. “Dual-mode and label-free detection of exosomes from plasma using an electrochemical quartz crystal microbalance with dissipation monitoring.” Analytical Chemistry, vol. 94, no. 5, 24 Jan. 2022, pp. 2465–2475, https://doi.org/10.1021/acs.analchem.1c04282. ↩︎
- Sebastiani, Federica, et al. “Screening of the binding affinity of serum proteins to lipid nanoparticles in a cell free environment.” Journal of Colloid and Interface Science, vol. 610, Mar. 2022, pp. 766–774, https://doi.org/10.1016/j.jcis.2021.11.117. ↩︎
- O’Bryan, Christopher S., et al. “Electrochemically deposited molybdenum disulfide surfaces enable polymer adsorption studies using quartz crystal microbalance with dissipation monitoring (QCM-D).” Journal of Colloid and Interface Science, vol. 614, May 2022, pp. 522–531, https://doi.org/10.1016/j.jcis.2022.01.098. ↩︎
- Rogala, Agnes, et al. “Using QCM‐D for Real‐time analysis of cell adhesion dynamics at Biointerfaces.” Advanced Materials Interfaces, 27 Nov. 2024, https://doi.org/10.1002/admi.202400811. ↩︎
- Moon, Suji, et al. “Unraveling how antimicrobial lipid mixtures disrupt virus-mimicking lipid vesicles: A QCM-D study.” Biomimetics, vol. 9, no. 2, 24 Jan. 2024, p. 67, https://doi.org/10.3390/biomimetics9020067. ↩︎
- Dunmyer, McKenna, et al. “Molecular scale adsorption behavior of per- and poly-fluoroalkyl substances (PFAS) on model surfaces.” Chemical Engineering Journal, vol. 497, Oct. 2024, p. 154286, https://doi.org/10.1016/j.cej.2024.154286. ↩︎
- Lee, Michelle C., et al. “Mitigating the astringency of acidified whey protein in proteinaceous high internal phase emulsions.” ACS Applied Bio Materials, vol. 3, no. 12, 15 Nov. 2020, pp. 8438–8445, https://doi.org/10.1021/acsabm.0c00767. ↩︎
- Karczmarczyk, Aleksandra, et al. “Development of a QCM-D biosensor for ochratoxin a detection in red wine.” Talanta, vol. 166, May 2017, pp. 193–197, https://doi.org/10.1016/j.talanta.2017.01.054. ↩︎