An aging population, injuries, and the prevalence of chronic diseases is leading to a growing demand for advanced therapies that can restore function and improve the quality of life. Traditional methods, such as organ transplants and prosthetics, often fall short due to limitations in donor availability and biocompatibility. The field of tissue engineering and regenerative medicine addresses the urgent need for innovative solutions to repair and replace damaged tissues and organs. Electrospinning has emerged as a promising technique in this context, offering the ability to fabricate highly customizable and biomimetic scaffolds. These scaffolds mimic the natural extracellular matrix (ECM), providing an ideal environment for cell growth, differentiation, and tissue regeneration. By wielding the potential of electrospinning, researchers are developing cutting-edge solutions that hold the promise of changing the field of regenerative medicine. Here we discuss a few of these areas, including skin regeneration, bone tissue regeneration, cardiac and vascular regeneration, and cellular therapies.
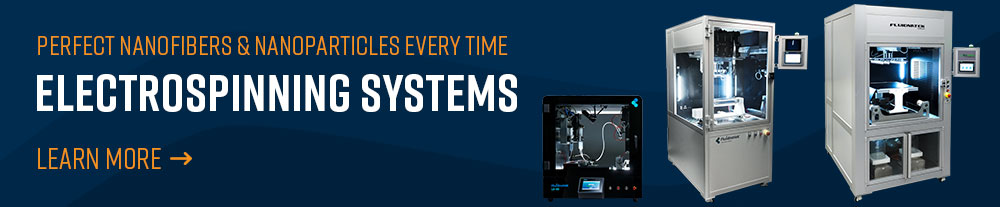
Skin Regeneration
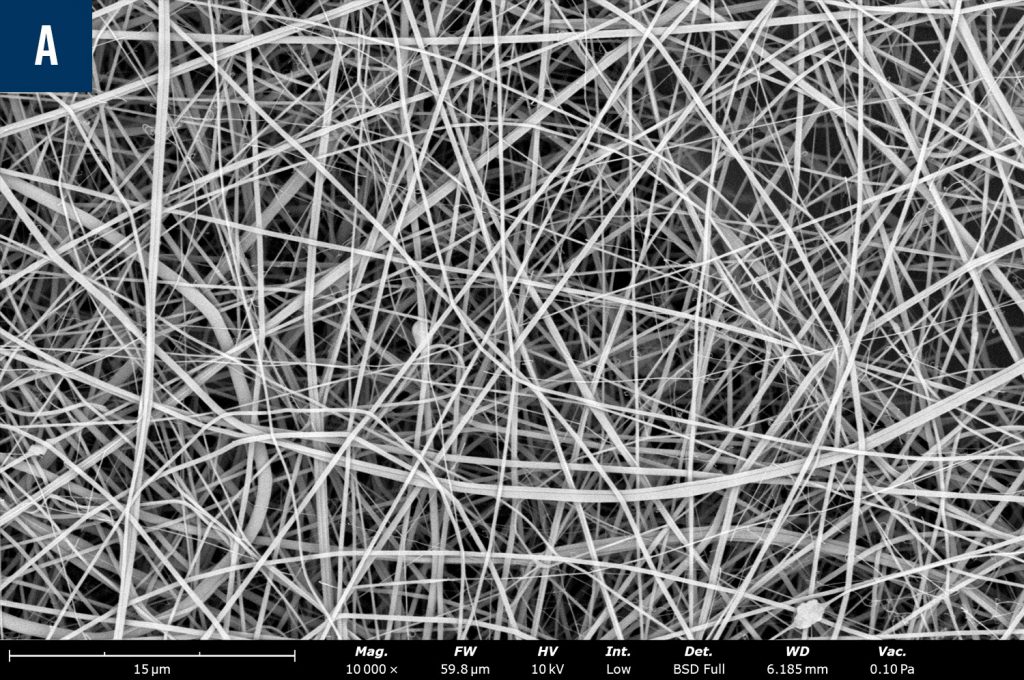
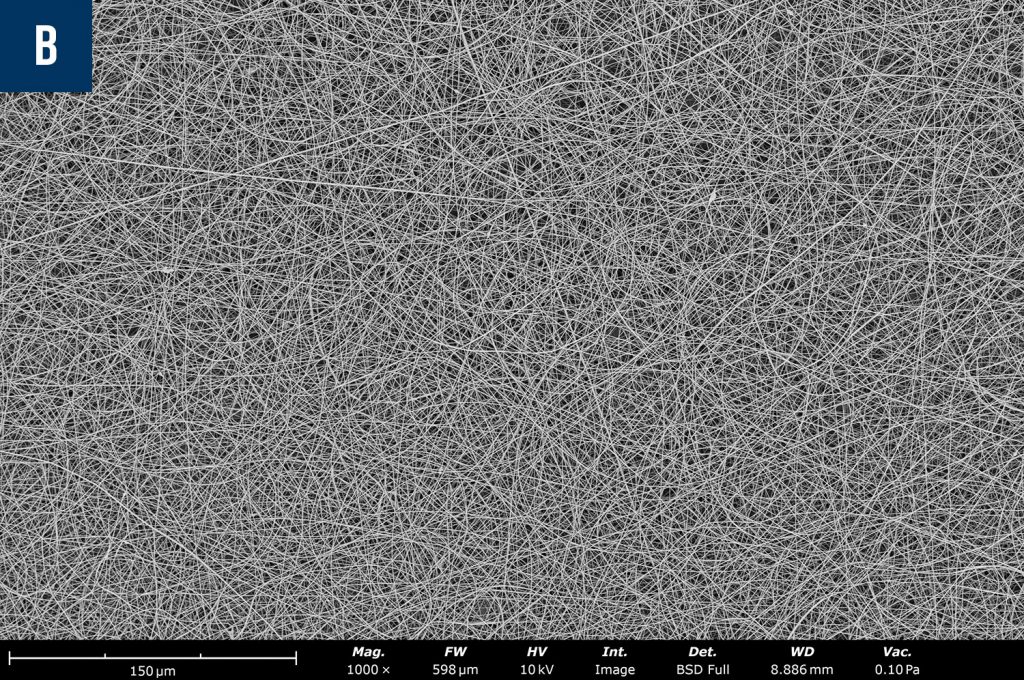
Electrospun scaffolds have shown great promise in skin tissue engineering. The usability of biologically relevant polymers from both natural and synthetic sources along with the excellent mimicry of the ECM allow the nanofibrous scaffolds to integrate well with the native tissues. For instance, scaffolds made from biopolymers like collagen and chitosan can support the growth of keratinocytes and fibroblasts, essential for skin repair and regeneration.1 Figure 1 show SEM images of collagen and chitosan electrospun nanofibers that are commonly used in skin tissue engineering. A recent study demonstrated that electrospun nanofibers applied to burn wounds significantly enhanced wound healing, suppressing inflammation and achieving uniformity of wound closure in animal models.2
Bone Tissue Engineering
Electrospinning has also been employed to create scaffolds for bone regeneration. These scaffolds often incorporate hydroxyapatite, a naturally occurring mineral in bone, to enhance osteoconductivity. Researchers have successfully used electrospun polycaprolactone (PCL)/gelatin (GEL) fibers combined with hydroxyapatite to support the growth and differentiation of osteoblasts, promoting new bone formation.3 PCL has also been used to generate three-dimensional (3D) nanofiber scaffolds with radially aligned orientation used for endogenous bone regeneration.4 These scaffolds were expanded to better resemble the native microstructure and promote cell proliferation and regeneration.
Orthopedic Tissue Engineering
Skeletal muscle injuries, especially where tendons are damaged can become challenging for the body and can be difficult to heal completely. Electrospinning has been shown to improve the healing process when electrospun fibers have been employed to regenerate the damaged tissue.5 For example, Rotium® is an electrospun bioresorbable wick used for rotator cuff repairs.6 It is made of polyglycolic acid (PGA) and polylactide-co-caprolactone (PLCL) and has been engineered to improve handling during implantation.
Cardiac & Vascular Tissue Engineering
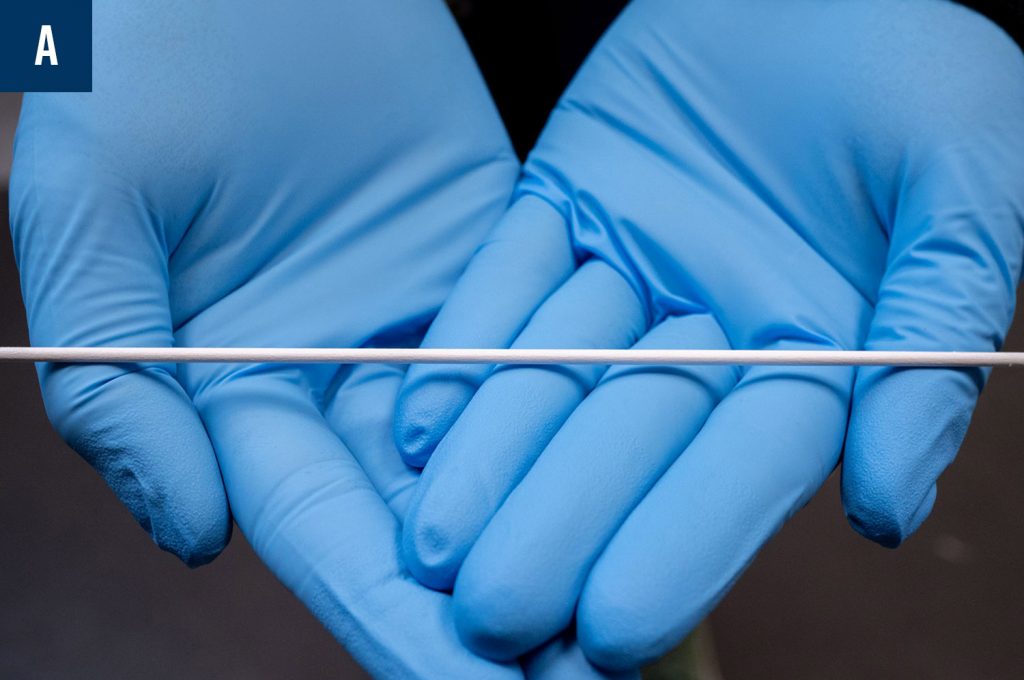
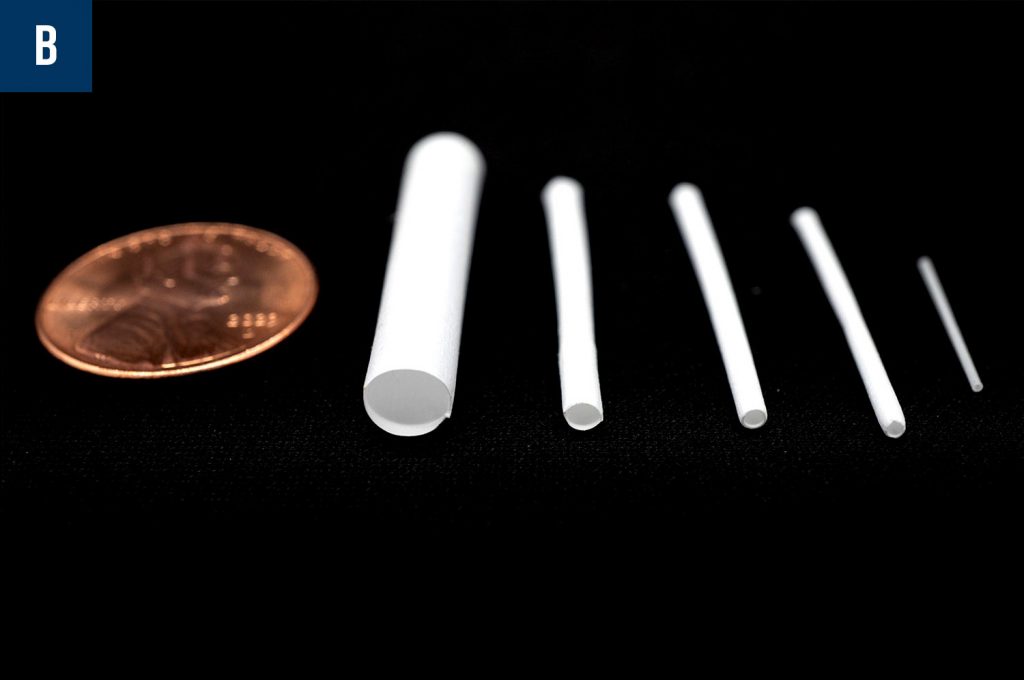
The development of electrospun scaffolds for cardiac tissue engineering aims to repair damaged heart tissue. Scaffolds made from materials like poly (lactic-co-glycolic acid) (PLGA) and gelatin have been used to support the growth of cardiomyocytes and vascular endothelial cells. Additionally, 3D nanofibrous scaffolds have been produced to replace damaged portions of cardiovascular/vascular tissues (Figure 2). These 3D constructs provide structural support and promote the growth and alignment of endothelial and smooth muscle cells, facilitating the regeneration of functional blood vessels and heart tissues.7
Nerve Regeneration
Electrospinning’s ability to create scaffolds that closely mimic natural tissue structures has led to exciting advancements in regenerative medicine. For example, researchers have developed electrospun scaffolds for nerve regeneration that guide the growth of neurons across injury sites. These scaffolds, often made from biodegradable polymers, provide a conducive environment for nerve repair.8 An example of this type of scaffolding can be seen in Figure 3.
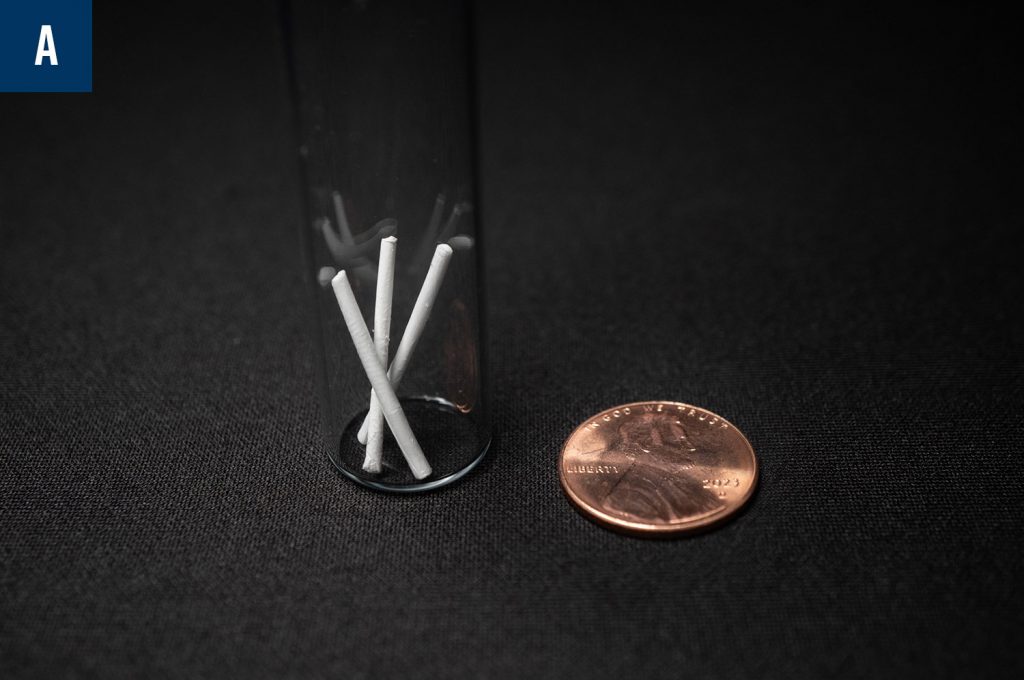
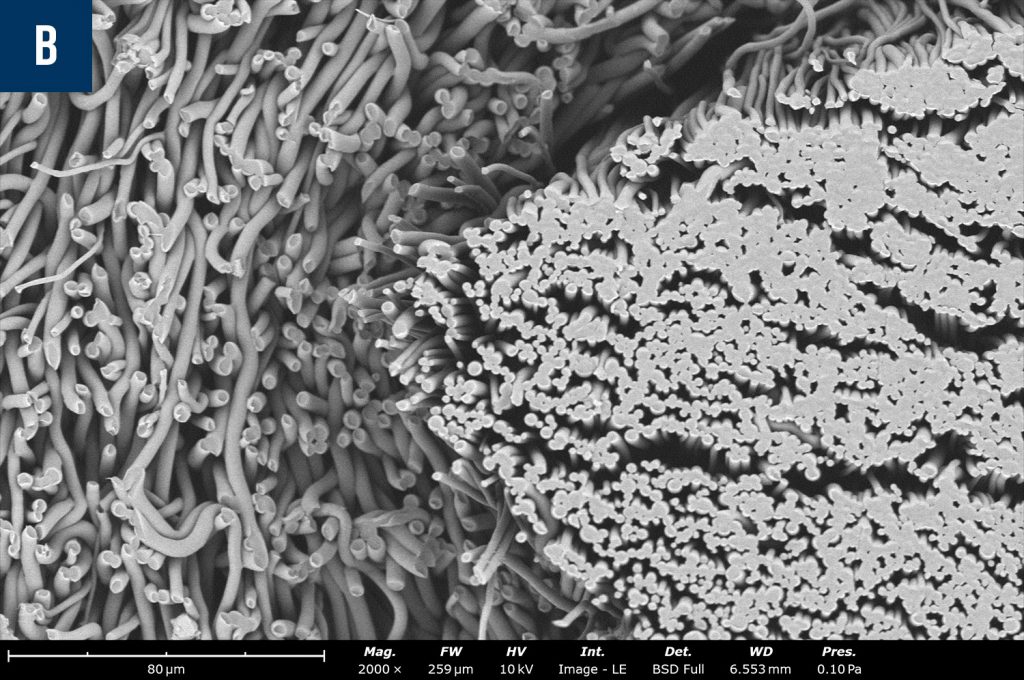
Research & Cellular Therapy
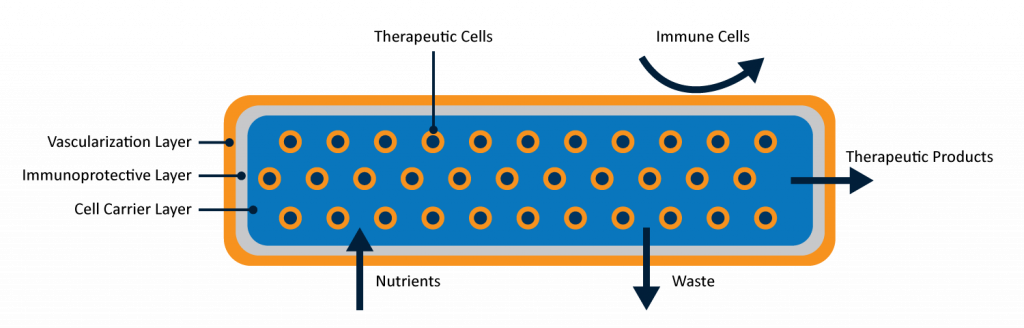
Electrospun materials are being explored for research and cellular therapy applications in regenerative medicine. For example, the Kalyptix® Immuno-protective Bilayer Membrane Technology by the Electrospinning Company addresses challenges of cellular therapies for disease treatment by providing an immuno-protective barrier offering promotion of cell growth, differentiation, and retention at the desired location (Figure 4). This is possible through the tunable nano and micro layers formed during the electrospinning and post-processing methods, as seen in Figure 4.9
Conclusion
Electrospinning is greatly impacting the field of tissue engineering and regenerative medicine with its ability to create highly customizable and biomimetic scaffolds. As research progresses, electrospun scaffolds are expected to play an increasingly critical role in developing advanced therapies for various tissue and organ regeneration applications.
References
- Wu, Jingwen, et al. “Electrospun nanofiber scaffold for Skin Tissue Engineering: A Review.” ACS Applied Bio Materials, vol. 7, no. 6, 22 May 2024, pp. 3556–3567, https://doi.org/10.1021/acsabm.4c00318. ↩︎
- Terezaki, Asimina, et al. “Ulvan/gelatin-based nanofibrous patches as a promising treatment for Burn Wounds.” Journal of Drug Delivery Science and Technology, vol. 74, Aug. 2022, p. 103535, https://doi.org/10.1016/j.jddst.2022.103535. ↩︎
- Liu, Jun, et al. “Polycaprolactone/gelatin/hydroxyapatite electrospun nanomembrane materials incorporated with different proportions of attapulgite synergistically promote bone formation.” International Journal of Nanomedicine, Volume 17, Sept. 2022, pp. 4087–4103, https://doi.org/10.2147/ijn.s372247. ↩︎
- Xiao, Lingfei, et al. “A radial 3D polycaprolactone nanofiber scaffold modified by biomineralization and silk fibroin coating promote bone regeneration in vivo.” International Journal of Biological Macromolecules, vol. 172, Mar. 2021, pp. 19–29, https://doi.org/10.1016/j.ijbiomac.2021.01.036. ↩︎
- Seetharam A, et. al. Use of a Nanofiber Resorbable Scaffold During Rotator Cuff Repair: Surgical Technique and Results After Repair of Small- to Medium-Sized Tears. Orthopaedic Journal of Sports Medicine. 2022;10(5). doi:10.1177/23259671221094848 ↩︎
- Rotium ® https://www.atreonortho.com/ ↩︎
- Han, Fengxuan, et al. “Performance of a multilayered small-diameter vascular scaffold dual-loaded with VEGF and PDGF.” Biomaterials, vol. 34, no. 30, Oct. 2013, pp. 7302–7313, https://doi.org/10.1016/j.biomaterials.2013.06.006. ↩︎
- Pramanik, S., Muthuvijayan, V. (2022). Electrospun Nanofibrous Scaffolds for Neural Tissue Engineering. In: Jayakumar, R. (eds) Electrospun Polymeric Nanofibers. Advances in Polymer Science, vol 291. Springer, Cham. https://doi.org/10.1007/12_2022_130 ↩︎
- https://www.electrospinning.co.uk/cell-therapy/ ↩︎