Microscopes have revolutionized our ability to observe and analyze the microscopic world with unprecedented detail. By enlarging our view of objects, or magnifying them, microscopes allow us to visualize a greater amount of detail and distinguish (or “resolve”) features separated by very small distances.
The scanning electron microscope (SEM) has quickly become one of the most broadly used microscopes in science and industry. Unlike optical microscopes which use visible light and lenses, SEMs use a focused electron beam and electromagnetic lenses. Using electrons instead of light is what allows electron microscopes, such as the SEM, to reach far greater resolving powers than light microscopes and provide information with a much greater scientific value.
At the heart of any microscopy technique lies two crucial concepts: magnification and resolution. While often used interchangeably, these terms represent distinct aspects of image formation and image interpretation. In this blog, we’ll delve into the definitions of magnification and resolution in the context of SEM, discuss their significance, explore their relationship, and consider their practical implications for sample analysis.
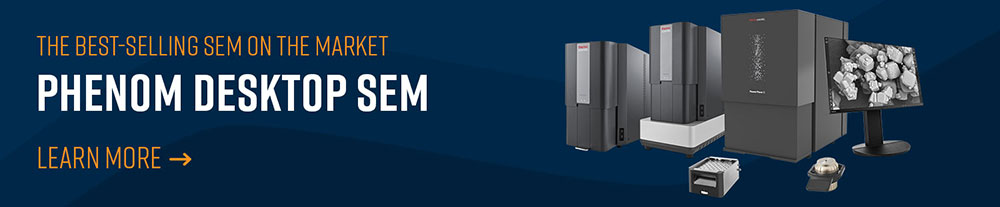
Magnification: Enlarging the View
Magnification refers to the process of enlarging the view of an object to see finer details. In SEM, the electron beam is raster scanned across a defined area on the sample surface and various signals are collected. At each X-Y position that the beam encounters, the detectors will record a signal intensity value which is used to reconstruct a black-and-white image on a digital monitor. In this way, an enlarged image of the object is formed which can be described by a magnification value. This value is often listed on the data bar in an SEM image.
Magnification is expressed as a ratio, comparing the apparent size of the specimen in the image to its actual size. In SEM, we can simplify this definition as the image length, Li, divided by the scan length, Ls. For example, a magnification of 5000x means that the image length is 5000 times larger than the scan length.
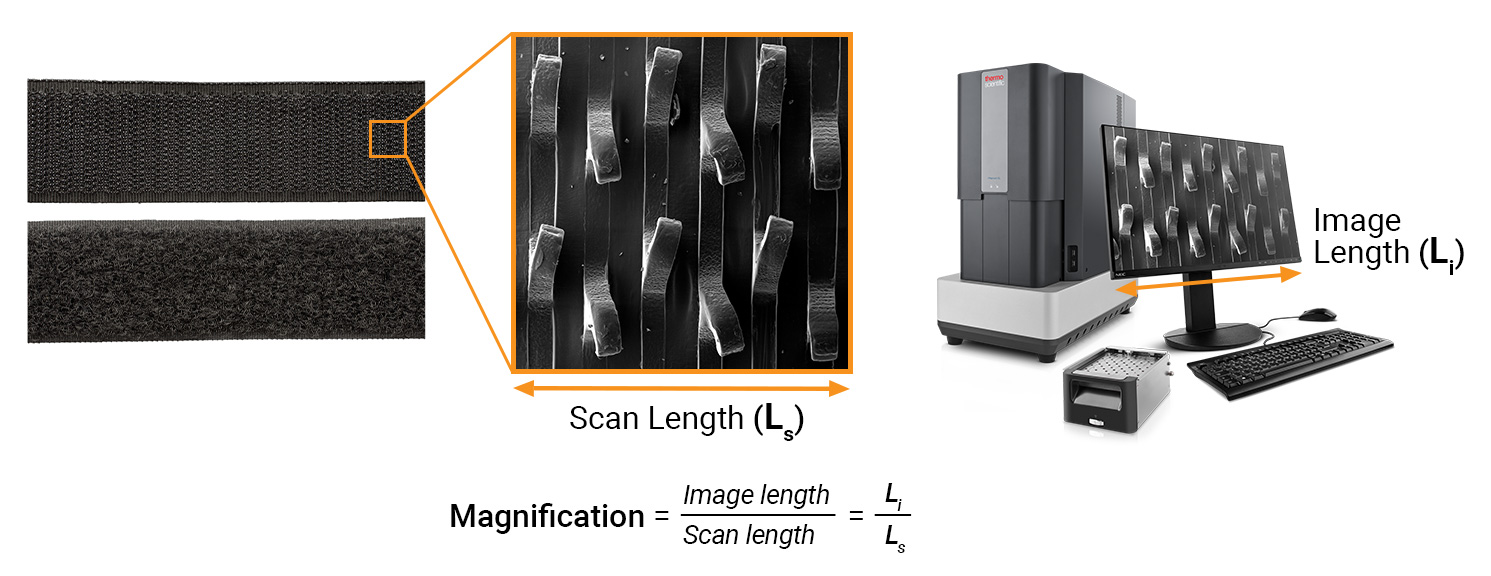
However, because the display size of an SEM image depends on the size of the digital monitor and the absolute size of the output image file, comparing magnification values between different SEM models can be misleading. Using the same magnification settings on different SEM instruments may result in different scan lengths and resulting images that appear to be at different magnifications. For this reason, magnification holds little scientific value when it comes to interpreting SEM images.
Some SEM systems can optionally display the polaroid magnification value. This gives the magnification value based on how big the image would be if it were printed on a polaroid (4 by 5 inches). While it’s not always exact, it can be a more objective way of comparing images from different systems. When comparing SEM images, it is always best to refer to the scale bar (or scan length), which is an accurate and reliable metric regardless of magnification value.
Resolution: Revealing Fine Details
Resolution refers to the ability of an imaging system to distinguish between closely spaced objects. Given sufficient light, our eyes can distinguish between points in space as small as 0.2 mm, or about the width of a strand of hair. Anything smaller than that would appear as a single object. For example, we cannot see individual plant cells within a leaf until we view it under an optical microscope. Using an SEM would allow us to dive deeper and visualize the cell walls and intracellular regions in greater detail.
The theoretical resolution of a microscope is expressed as the minimum distance between two distinct points that can still be distinguished in an image (i.e., Rayleigh criterion). Smaller resolution values indicate a higher resolving power. Image formation is diffraction-limited meaning the incident radiation (be it light or electrons) will blur the image of the object due to the bending of waves as they pass around the edge of an object. How much distortion, or blurring, depends upon the wavelength of the incident radiation.
A general rule to follow is that the resolution is limited to half of the incident wavelength. Visible light has a minimum wavelength of ~400 nm which means that the resolution of a light microscope is limited to about 200 nm. On the other hand, the wavelength of high-energy electrons that are accelerated toward a sample in an SEM is thousands of times smaller – on the order of picometers (10-12 meters). This is why the resolving power of electron microscopes is so much better than light microscopes.
How is resolution measured in SEM?
Resolution is a key metric often used to rank the capability of different SEM instruments. While the Rayleigh criterion is a theoretical limit used to describe diffraction-limited resolution, it cannot be applied to real SEM instruments because several technical factors (e.g., lens imperfections, environmental vibrations, etc.) degrade resolution. In practice, the actual resolution of an SEM can range from 1 to 20 nm.
Edge resolution (or knife edge resolution) is typically used to quantify the resolution of actual SEM systems. Knife edge resolution measures the distance between a “white” point and a “black” point from an intensity profile drawn perpendicular to an edge feature, where the definition of white and black are defined by arbitrary intensity values. Depending on the definition of white and black, the resolution measure can change significantly. Because edge resolution is not a standardized metric, it’s always important to understand how it is being reported. The best way to get an idea of a system’s resolution is to get a live demonstration and see it for yourself.
The Relationship between Magnification and Resolution
While magnification and resolution are distinct concepts, they are closely related in SEM. Increasing magnification often leads to the perception of higher resolution, as finer details become more apparent. However, it’s important to note that magnification alone does not guarantee improved resolution. As a general rule of thumb, the minimum feature size you can measure with a reasonable margin of error is ~10 times the resolution, so at 10 nm resolution you can measure 100 nm particles.
Achieving high resolution requires not only increasing magnification but also optimizing the SEM settings, such as beam intensity, accelerating voltage, and detector configuration. Additionally, factors like sample preparation and specimen conductivity can influence the resolution of SEM images.
Considerations for Sample Analysis
When using SEM for sample analysis, it’s crucial to consider both magnification and resolution to obtain meaningful results. Here are some key considerations:
Balancing Magnification and Resolution:
Striking the right balance between magnification and resolution is essential. While higher magnification levels may provide more detailed images, they can also lead to decreased depth of field and increased noise. It’s important to choose magnification settings that optimize both resolution and field of view for the specific features of interest.
Sample Preparation:
Proper sample preparation is critical for achieving high-resolution SEM images. Samples must be appropriately mounted, cleaned, and coated (if necessary) to minimize charging effects and ensure optimal conductivity. Additionally, selecting the appropriate imaging parameters and techniques can enhance resolution and reveal finer details – for more info see our blogs on low-voltage SEM and how to reduce sample charging.
Understanding Instrument Limitations:
SEM instruments have inherent limitations in terms of magnification and resolution. It’s essential to understand the capabilities and limitations of the specific SEM system being used and adjust expectations accordingly. One of the major factors in determining SEM resolution is the type of electron source it is using – for more info check out our blog comparing different types of electron sources. Collaborating with experienced microscopy experts can help navigate these challenges and maximize the quality of the SEM data.
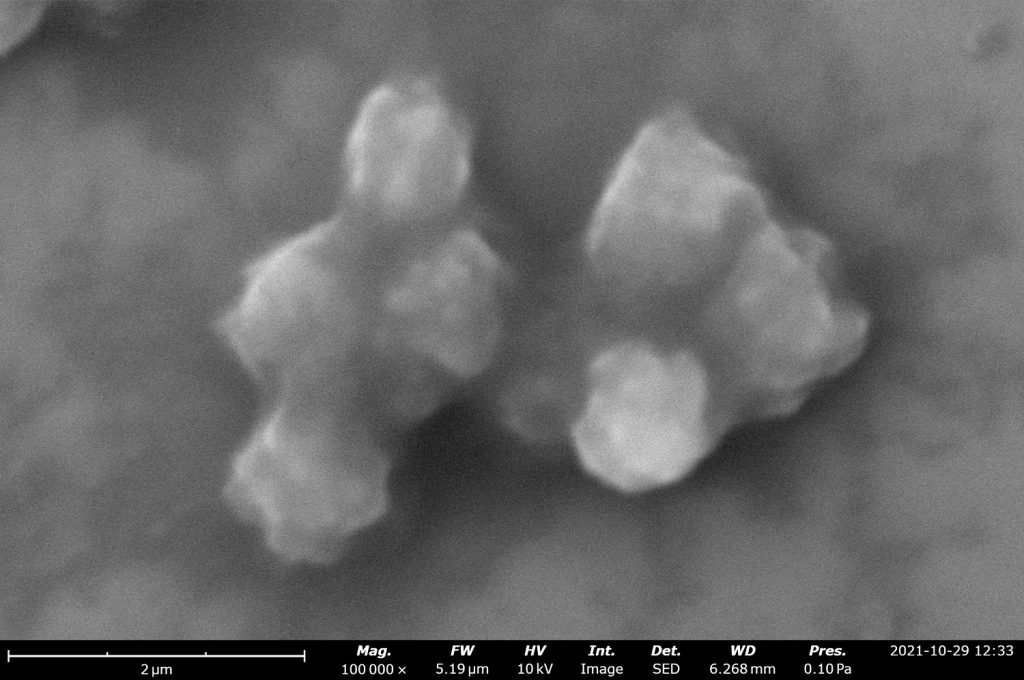
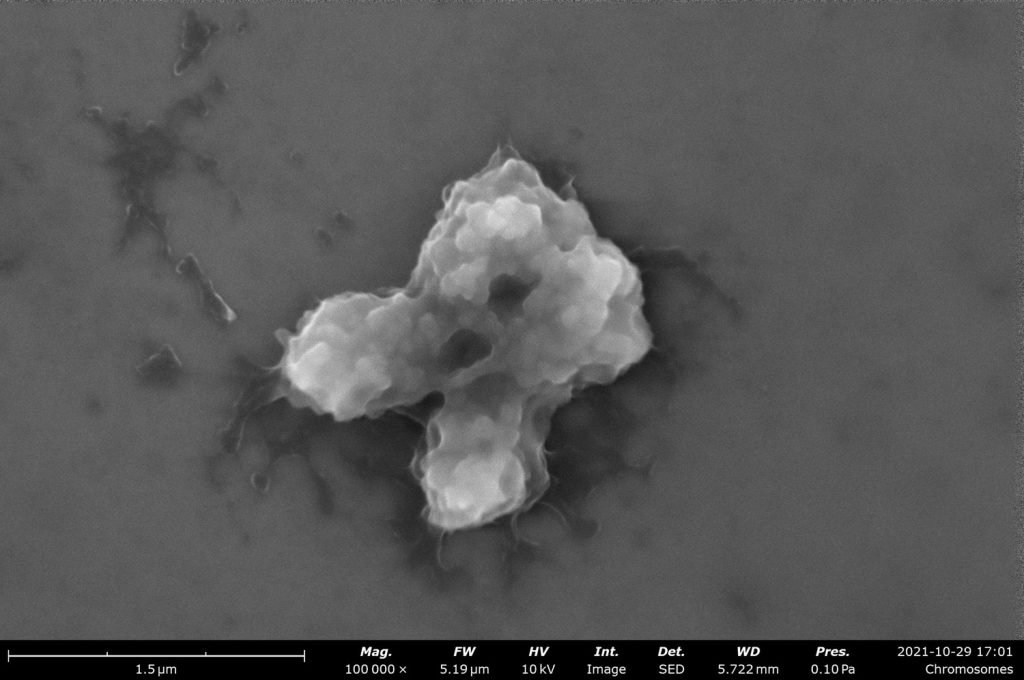
Key Takeaways
In conclusion, magnification and resolution are fundamental concepts in SEM, each playing a unique role in image formation and interpretation. While magnification refers to enlarging the view of the specimen, resolution is a quantitative description of the capability of a microscope to visualize small features. Understanding the relationship between magnification and resolution and considering their implications is essential for effectively utilizing SEM in sample analysis and research. By carefully optimizing imaging parameters and techniques, researchers can harness the full potential of SEM to uncover meaningful insights.