What is Impedance?
Impedance is the frequency-dependent resistance of a circuit or electrochemical system to current flow. Applying a small AC sine wave perturbation to the electrochemical system and measuring the resulting response makes it possible to analyze how the system’s electrical properties vary with different frequencies or timescales. The resulting impedance spectrum provides information about the various electrochemical processes, such as charge transfer reactions, mass transport phenomena, and diffusion processes.
Valid impedance spectroscopy (IS) data requires a system to be stable, causal, and linear. Since only a small sine wave signal is applied to the system under test, it ensures a linear response is achieved. Impedance spectroscopy (IS) is a non-destructive technique which makes it an attractive technique for measuring many different electrochemical systems leaving them unaffected at the end of the experiment.
Once impedance data has been collected, it needs to be analyzed to extract the information contained in the result. The main way this is achieved is through equivalent circuit modeling. Electrochemical systems behave like electrical circuit elements and a network of elements can be built to be fitted to the data. In addition to the standard circuit elements like resistors, capacitors, and inductors, electrochemists have introduced additional elements to help to understand the information.
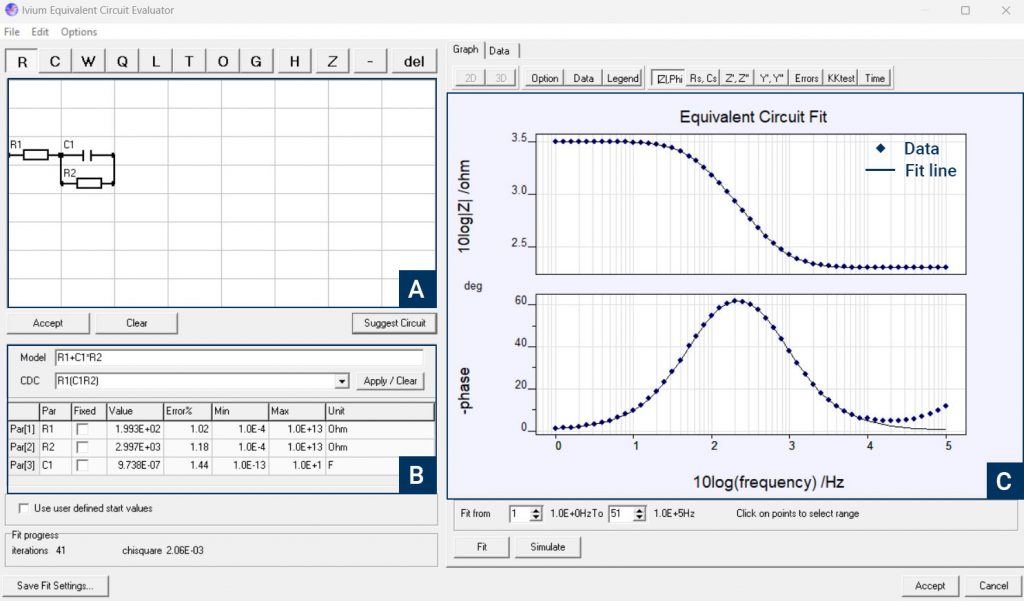
How can impedance be used for battery testing?
Batteries are complicated devices made up of a positive electrode (cathode), a negative electrode (anode), a separator, and an electrolyte. All these components contribute to the performance of the battery. Standard DC testing can have difficulty in separating the responses resulting from the constituent parts. Since impedance is measured over a wide range of timescales (frequencies), it can probe the responses from different parts of the battery.
The impedance of lithium-ion batteries is a complex quantity that depends on various processes and parameters within the cell. Some of the information that is extracted includes:
- Resistance inside the battery, both ohmic and charge transfer.
- Double-layer capacitance which relates to the charge storage capability of the battery.
- Distinguishing between capacitive behavior and faradaic processes to understand the reaction kinetics.
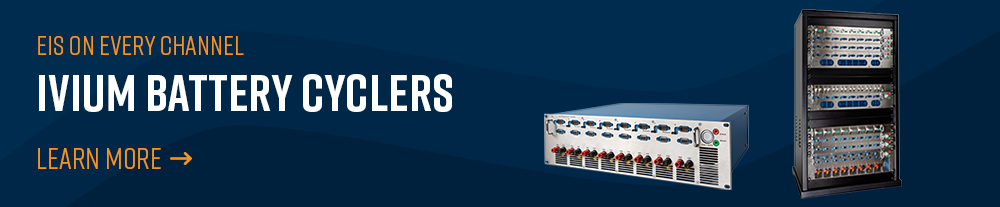
Conductivity:
The conduction properties of battery materials have been investigated with impedance (1). Different approaches have been used to examine the ionic and electronic conductivity of cathode (positive electrode) materials.
Ion and electron-blocking setups have been created using circular pellets of cathode materials. For an ion-blocking setup, metal electrodes are evaporated onto the 2 parallel faces of the pellet and the metal electrodes only conduct electrons to measure the electronic conductivity. In the case of electron electron-blocking setup, a polymer electrolyte layer is placed in between the metal electrodes and the pellet. Only ions can cross the electrolyte layer, blocking the movement of electrons. The ionic conductivity is at least an order of magnitude lower than the electronic conductivity indicating that the conduction of lithium ions is rate-limiting. Similar methods have been used to study solid electrolytes. By employing a combination of different cell setups (ion blocking, electron blocking, and reversible) impedance arcs in the Nyquist plot could be attributed to ionic, bulk, and interfacial contributions.
Temperature:
The effect of the temperature of the battery on its impedance has been studied, as it affects the internal resistance and the electrochemical reaction speed of the cell [2]. At low temperatures, the impedance increases due to higher resistances and lower reaction rates, resulting in lower capacity and power output. At high temperatures, the impedance may decrease due to lower resistances and faster reaction rates. Increased temperature may also increase the risk of thermal runaway and degradation. Crystalline solid electrolytes have been studied with the possibility of separating grain and grain boundary contributions to the impedance measurements [3].
Battery Aging:
Impedance measurements have also been used to study the aging of lithium-ion batteries, both due to the cycling of the batteries and calendar aging [4]. Impedance results show that as the capacity of the battery fades during cycling, the main increase in impedance is due to the cathode material which undergoes particle cracking. This cracking can increase the impedance by isolating parts of the electrode as well as consuming some of the electrolyte.
Solid Electrolyte Interface (SEI):
A dramatic increase in the impedance at the SEI can be observed under elevated temperatures. This can be attributed to an increase in the thickness of the SEI layer because of increased reactivity.
State of Health (SOH) EIS can reveal changes in electrochemical behavior which helps to identify degradation mechanisms such as electrode aging, electrolyte degradation, formation of solid-electrolyte interphase (SEI) layers, and changes in ion transport properties—monitoring changes in the resistances (impedances) in the battery yields aging and degradation progression.
Conclusion
Electrochemical impedance spectroscopy (EIS) is a powerful technique for understanding the constituent parts of lithium-ion batteries. It can be used to comprehend the predominant electronic and ionic mechanisms at the positive and negative electrodes and through the separator. This then allows the aging processes to be elucidated and methods developed to benchmark the state of health of a battery.
References
[1] Amin R. et al., J. Electrochem. Soc., 163 (8), A1512 (2016)
[2] Jalkanen K. et al., Applied Energy, 154, 160 (2015)
[3] Riegger L. et al., Angew. Chem. Int. Ed., 60 (12) 6178 (2021)
[4] Waag W. et al., Applied Energy, 102, 885.