What is a potentiostat?
A potentiostat is used to maintain a constant potential at the working electrode in a 3-electrode electrochemical cell and is the principal piece of equipment used in electrochemical experiments. The potential difference between the reference electrode and the working electrode is maintained by a feedback mechanism where the potential of the counter electrode is adjusted accordingly. This adjustment happens at very high speeds, so the potential is typically achieved instantaneously. In addition, most potentiostats also function as galvanostats where the current is controlled instead of the potential.
For many users, potentiostats are black boxes that they connect to their electrochemical cell and measure current and voltage. A basic understanding of a potentiostat’s functioning can help when troubleshooting unexpected results in experiments.
Components of a Potentiostat
The main component of a potentiostat system is the potentiostat box containing the control electronics. The essential electronic circuitry inside the potentiostat box is an operational amplifier (op-amp). An Op-amp is a voltage-amplifying device with a differential input and a single-ended output. It is used to amplify weak electric signals. In a potentiostat, op-amps are used to control the voltage and current flowing in the electrochemical cell and are responsible for maintaining the voltage between the working electrode and reference electrode as close as possible to the desired voltage.
Op-amps typically have high amplification factors or gains. For practical purposes, this means that the voltage difference at the inputs must be very small. For example, an op-amp with a gain of 106 must keep the voltage difference at the inputs below 0.015 mV for a 15 V supply voltage.
A negative feedback loop, where the output voltage is fed back to one of the inputs, minimizes the difference at the input.
The op-amp works by controlling the voltages at the 2 inputs to be equal. It achieves this by adjusting the counter electrode voltage (output voltage).
Figure 1 represents the schematic of the connections in the electrochemical cell. The solution resistances in the cell between the counter electrode and the reference electrode, and between the reference electrode and the working electrode, represent a voltage divider. Hence, the voltage of the reference electrode vs. the working electrode is controlled and known.
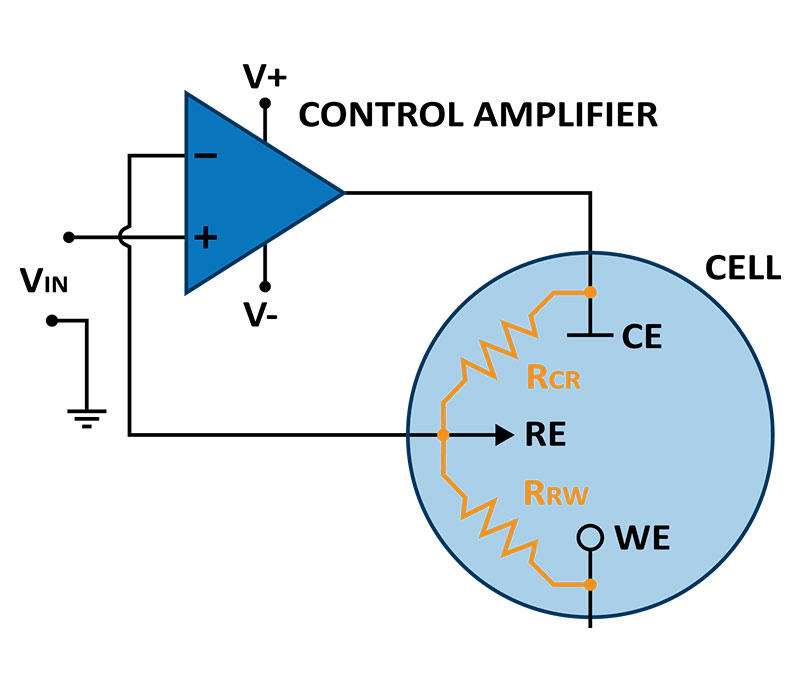
Signal generation and measurement
The second no less important part of the potentiostat electronics is the signal generation and measurement component. This is the part that generates the applied waveforms and converts the voltage and current observed in the cell into measurable signals that can be transferred to the computer for recording and presentation. Modern potentiostats perform this using digital-to-analog (DAC) and analog-to-digital (ADC) converters. DAC/ADC Converters divide smooth analog signals into discrete chunks called bits. While this enables the generation of complex waveforms, a downside is that bit resolution can cause waveform distortion and misinterpretation of the data. The data can also be passed through some signal conditioning steps to filter out noise, which can lead to unexpected offsetting of data due to asymmetric noise signals. The user needs to be aware of these possibilities when interpreting unexpected results.
Cell connection cable
The cell cable is a bundle of individual cables that form part of the system that connects the potentiostat to the electrochemical cell. Typically, the connections for the current-carrying cables and the voltage-sensing cables are separated to reduce voltage drop and contact resistance effects. The working electrode is connected to a red current lead and a blue voltage sensing lead, the reference electrode is connected to a white reference lead, and the counter electrode is connected to a black current-carrying lead. The cell cable may also include a ground lead (green).
Why use potentiostats?
Why use a potentiostat instead of a multimeter and current/voltage source? The primary reason for using a potentiostat is that it can accurately control a single electrode in a multi-electrode electrochemical cell. In a three-electrode electrochemical cell, the potentiostat controls the potential between the working or sample electrode and the reference electrode. It measures the current between the working electrode and the counter electrode. No current (or very little current) flows through the reference electrode, allowing it to maintain a constant potential. In this way, the electrode of interest is isolated from the other electrodes and its current-voltage behavior can be studied. Figure 2 illustrates the arrangement of the electrodes and where the current and voltage are measured.
![Figure 2: Schematic representation of an electrochemical cell connected to a power source, indicating the points where voltage and current are measured [1].](https://www.nanoscience.com/wp-content/uploads/2023/09/electrochemical-cell-connected-to-power-diagram.jpg)
Conversely, in a 2-electrode arrangement, only the current-voltage behavior of the whole cell can be probed. This includes both electrode interfaces and the solution between the 2 electrodes.
Common Potentiostat applications
Corrosion
Corrosion and corrosion prevention are a large global annual cost, totaling over $3 trillion a year (based on NACE 2016 estimate of $2.5 trillion). An example of this cost can be seen in the collapse of a bridge in Pittsburgh in 2022, the city with the largest number of bridges and tunnels in the US. Fortunately, no one was killed, but the replacement bridge could take years to build and far exceed the cost of timely prevention.
Electrochemical corrosion measurements can be non-destructive and are used to measure the corrosion rate inside pipelines, the integrity of painted metal surfaces, corrosion of the reinforcing bars in concrete structures, as well as laboratory studies of the evaluation of chemicals for the inhibition of corrosion and new materials for inclusion in building materials.
One of the most common experiments for measuring corrosion rates is the polarization resistance experiment. This is a non-destructive technique that polarizes the sample by a small amount on either side of the corrosion potential (the resting potential of the sample in solution). The corrosion rate can be determined by measuring the slope of the current-voltage curve. Other corrosion experiments are more destructive and are designed to qualitatively assess the corrosion behavior of materials. These include potentiodynamic polarization and Tafel experiments, as well as cyclic polarization.
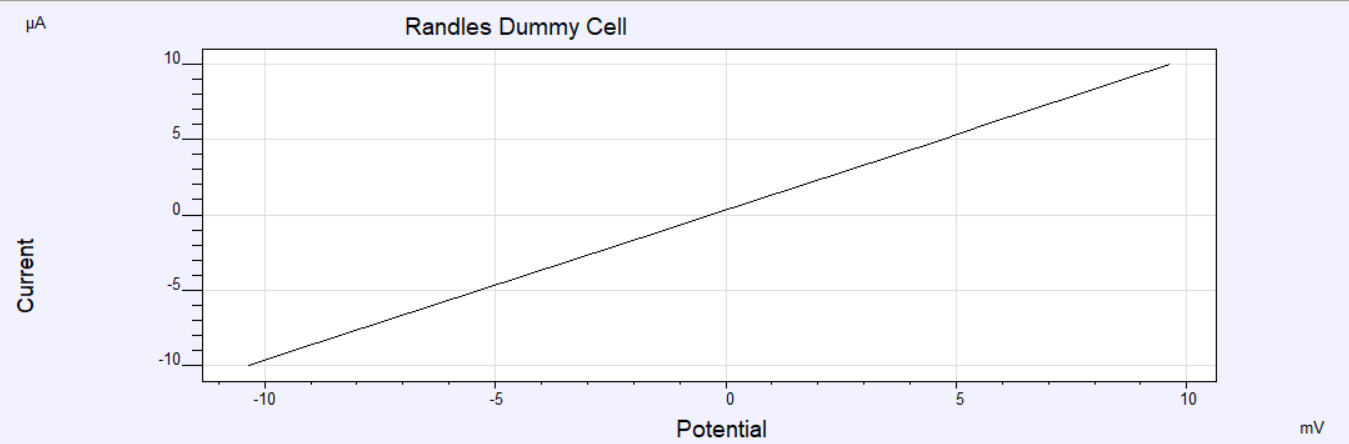
Organic Coatings
Organic Barrier Coatings are used as a physical corrosion prevention method in many applications including steel bridges, building cladding, paints on cars, soda can linings, and food cans. Electrochemical techniques are ideally suited to measure the integrity of coatings because the large difference in the dielectric constants of the paint and water means that small changes in the coating can be readily detected.
Electrochemical Sensors
A sensor is a device that detects a stimulus and transmits an electrical signal as a means of measuring the change of an intrinsic property. Electrochemical sensors are a class of chemical sensors in which an electrode is used as a transducer element in the presence of the quantity to be detected. Some areas of application for sensors are in medical diagnostics such as blood glucose meters, gas sensors in the automotive industry, and in-home carbon monoxide detectors. Many diagnostic medical tests are performed with electrochemical sensors, like ion-selective electrodes. Heavy metal ion sensors are also used to investigate water quality.
Battery and Battery Materials Testing
Battery materials testing is a crucial aspect of the development and optimization of battery technologies. It involves evaluating the properties and performance of materials used in batteries, such as electrode materials, electrolytes, separators, and current collectors. The goal of battery materials testing is to understand and improve the efficiency, capacity, safety, and overall lifespan of batteries. Battery materials testing is an iterative process, involving the continuous refinement of materials based on test results and feedback. As new materials are developed and tested, the goal is to achieve higher energy densities, longer lifespans, and enhanced safety for batteries.
Electrochemical testing is suitable for testing the different components that make up the battery. Electrode materials, such as cathodes and anodes, are tested for their capacity, energy density, voltage profiles, and cycling stability. Performance under various charging and discharging rates, temperature ranges, and cycling conditions is assessed to understand their practical application in different scenarios. Structural stability and the ability to accommodate ion insertion/extraction during cycling are evaluated to prevent degradation.
For electrolytes and separators, the main concerns that are addressed by electrochemical measurements are ionic conductivity and chemical and electrochemical stability. Other non-electrochemical properties are also important.
Current collectors (usually made of conductive materials like copper or aluminum) are tested for their electrical conductivity and resistance to corrosion. Good electrical contact with electrode materials is essential for efficient charge and discharge cycles.
The batteries themselves are subjected to repeated charge and discharge cycles to simulate real-world usage conditions. Impedance tests can also yield valuable information about the parts of the battery that are affected most by repeated cycling. Aging tests involve subjecting batteries to prolonged exposure to elevated temperatures and various charge/discharge conditions to understand their long-term stability and performance.
Fuel Cells and Electrolyzers
High-performance fuel cells and electrolyzers are an important part of the green economy that is necessary to reduce our reliance on fossil fuels. They require state-of-the-art materials to achieve high efficiency. Electrochemical tests are used to design the electrode materials and separators that make up these systems. In addition, electrochemical tests like electrochemical impedance spectroscopy can help maintain the performance for enhanced durability.
Bioelectrochemistry
Bioelectrochemistry is a branch of electrochemistry and biophysical chemistry. It deals with electrophysiological topics like cell electron-proton transport, cell membrane potentials, and electrode reactions of redox enzymes. Typically, small currents and voltages are measured which requires specialized equipment that can handle these conditions.
Electrosynthesis
Electrosynthesis has always been an important part of the production of some chemicals but has recently come to the fore because of the advantages of improved selectivity and yields and reduced waste. Hazardous reagents may also be reduced, being replaced by electric current. Coulomb counting can also be used to identify the endpoint of a specific reaction.
Supercapacitors
Supercapacitors are high-energy capacitors that can store large amounts of energy by utilizing high surface area materials. Electrochemical tests can be used to identify suitable materials and evaluate performance under exacting conditions. Supercapacitors are required to deliver their energy in a very short period which can be evaluated with short-time pulses or impedance spectroscopy.
Photovoltaics
Energy generation from the sun is an important part of the renewable energy landscape. High-efficiency solar cells are being developed to extract more energy from the same area coverage. Much effort has been focused on Dye-sensitized solar cells which contain light-adsorbing molecules that convert the sun’s energy into electrical energy. Electrochemical tests have been instrumental in pushing this research area forward. Electrochemical impedance spectroscopy has helped to understand the reactions occurring in the solar cell.
References
[1] A. W. Colburn, K. J. Levey, D. O’Hare, and J. V. Macpherson, “Lifting the lid on the potentiostat: a beginner’s guide to understanding electrochemical circuitry and practical operation,” Physical Chemistry Chemical Physics, vol. 23, no. 14, pp. 8100–8117, Jan. 2021, doi: 10.1039/d1cp00661d.